HEDS Research Area
Fusion Science
- Home
- Research
- Research Areas
- Fusion Science
LLNL scientists conduct a broad range of fusion science research, including research focused on magnetic fusion energy, inertial confinement fusion experiments, and inertial fusion energy. Their work also includes efforts to develop new instrumentation and diagnostic technology that supports fusion science research. Many of LLNL’s fusion science experts take leadership roles in multi-institutional fusion research centers and initiatives, accelerating our pursuit of fusion-based energy.
Dive deeper into our research
Inertial Confinement Fusion (ICF)
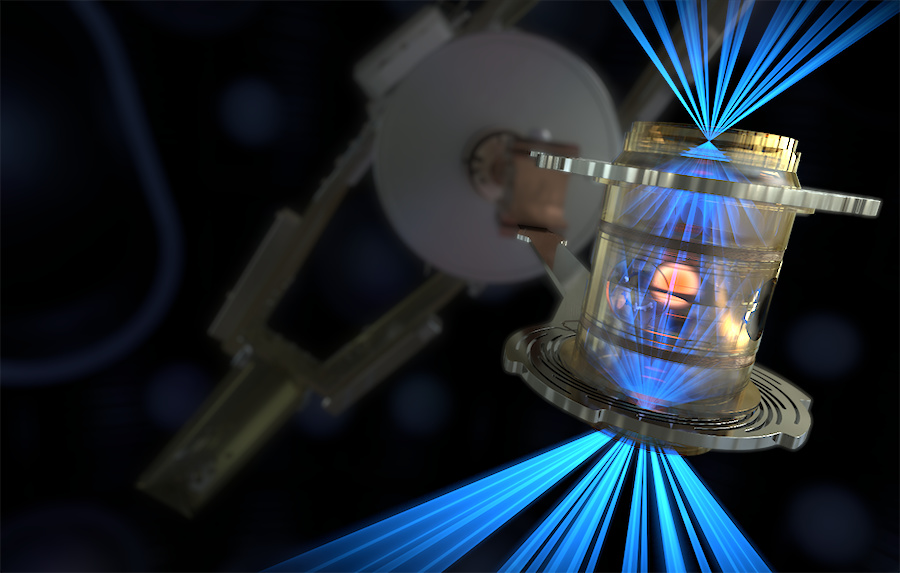
LLNL scientists explore the potential of achieving inertial confinement fusion (ICF) through an indirect-drive approach. Nuclear fusion reactions combine two light nuclei into a heavier one, releasing substantial energy in the process. In order to generate a significant level of reactivity, the fusion fuel must be at extremely high ion temperature and pressure, and it must be held in that state for a finite amount of time. The fundamental challenge of fusion is that while fusion reactions can self-heat the plasma, at high temperatures the plasma also rapidly loses energy via cooling mechanisms, such as x-ray emission and electron heat conduction.
The key element of any ICF design is the implosion of small capsule filled with fusion fuel. The indirect-drive approach to ICF uses lasers interacting with a high atomic number cavity (a “hohlraum”) to produce an intense bath of x rays. The capsule is placed at the center of the hohlraum and the outer surface, the ablator, absorbs the x-ray energy in a thin layer of ionized plasma generating an ablation pressure that drives the capsule inward, accelerating the fusion fuel to high velocity (the “implosion”).
The implosion compresses the fusion fuel to high temperature and pressures, by converting kinetic energy into pdV work, over a period determined by the inertia of the fuel itself. If the conditions are right, a positive thermodynamic feedback loop is created in the fusion fuel that “ignites” the plasma, generating an explosive, microscopic-scale release of energy. Achieving the conditions required for ignition requires an incredible degree of control over several physical processes and engineering aspects of the target.
Learn More
- Celebrating the Milestone of Ignition
- LLNL’s Breakthrough Ignition Experiment Highlighted in Physical Review Letters
- Ignition gives U.S. ‘unique opportunity’ to lead world’s IFE research
Contact
- Omar Hurricane
- hurricane1 [at] llnl.gov (hurricane1[at]llnl[dot]gov)
Magnetic Fusion Energy (MFE)
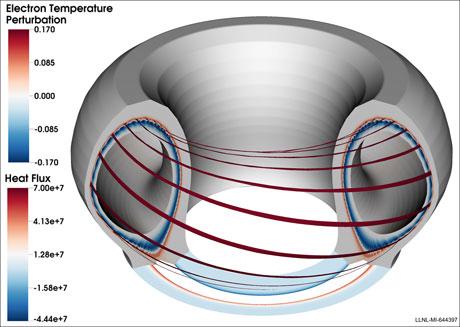
LLNL physicists are involved in multiple aspects of magnetic fusion energy (MFE) research, including fusion/plasma system modeling and performance optimization, as well as development and field testing of instrumentation and diagnostics for fusion energy experiments. For example, they compare experimental results with analytical theory and computational models of plasma behavior, develop novel diagnostic measurement techniques, and execute state-of-the-art MFE experiments focused on both the core and boundary of high-performance MFE plasmas.
These advanced diagnostics are relevant to some of the most ambitious energy projects in the world, including the multi-nation ITER magnetic fusion device project, the world's largest tokamak, which is being built in France. LLNL scientists played a key role in magnet design for the ITER tokamak. In addition, LLNL scientists contribute to experiments conducted at the DIII-D National Fusion Facility, located in San Diego, California, and operated by General Atomics for the U.S. Department of Energy (DOE). The aim of this project is to establish the scientific basis for the tokamak approach to fusion energy production.
LLNL scientists also are involved in experimental work conducted at the National Spherical Torus Experiment-Upgrade (NSTX-U) fusion facility at DOE’s Princeton Plasma Physics Laboratory. A key research object at NSTX-U is to explore the capability of the spherical facility to produce stable, high-performance plasmas with low-cost magnetic fields.
In addition, LLNL researchers are involved in plasma theory and computations in support of fusion experiments. They take advantage of LLNL’s advanced algorithm development and high-performance computing resources to simulate various kinetic and fluid plasma models. Their fusion modeling work also supports efforts to develop next-generation U.S.-based tokamaks.
For example, LLNL scientists are focused on developing integrated simulation capabilities to understand the physics of particle and energy fluxes onto material surfaces, under various conditions, such as plasma disruptions in large-scale MFE experimental facilities and future fusion power plants. The rapid advance of artificial intelligence and machine learning is already playing a growing role in the design of next-generation fusion power plants.
Learn More
- LLNL research team develops infrared camera system to view tokamak from the inside
- Physics Research Groups (see Fusion Sciences tab)
Contact
- Harry McLean
- mclean1 [at] llnl.gov (mclean1[at]llnl[dot]gov)