The Center invites HED science researchers to deliver presentations as part of our weekly seminar series for LLNL staff, postdocs, and interns.
All presentations are the work of the speakers and owned by their respective institutions. We thank the speakers for permission to post their work here. To watch featured seminars, please visit the Livermore Lab Events YouTube channel.
If you are interested in delivering a seminar, we invite you to contact Federica Coppari, seminar series chair, at coppari1 [at] llnl.gov (coppari1[at]llnl[dot]gov)
Geun Woo Lee
Korea Research Institute of Standards and Science/University of Science and Technology, Republic of Korea
Abundant H2O phases fo...
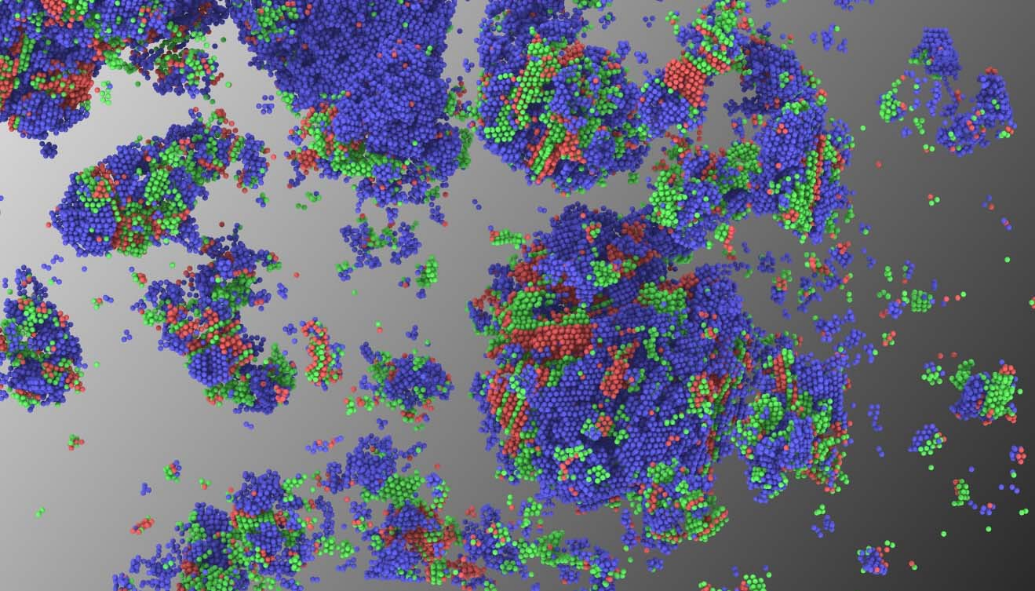
Geun Woo Lee
Korea Research Institute of Standards and Science/University of Science and Technology, Republic of Korea
Abundant H2O phases form due to various configurations of hydrogen-bonded networks under wide ranges of temperature and pressure. In particular, at low temperature, the slow kinetics of the cooperative motion of H2O molecules in the hydrogen-bonded networks can increase the chances that an ice phase takes place at deep metastable states across its equilibrium phase boundaries with high density and high bonding energy. Circumstantially, the most effective way to reduce the total Gibbs free energy of the ice is to create metastable phases which have slightly less energy than the ice. This process produces complicated transition pathways. Therefore, finding metastable ice phases are of importance to understand the phase transition mechanism.
However, such metastable phase formations and complicated transition pathways have been rarely observed at near and above room temperature, where thermodynamics is dominated for the phase transitions. Nevertheless, recent studies have raised the possibility to find metastable phases at room or high temperature, when deep metastability (or large driving force) can be achieved. Such examples are metastable ice VII in the stable regime of ice VI, high-density amorphous (HDA) at room temperature, and plastic ice VII at high temperature. In this regard, the ice crystallization due to the high degree of metastability and fast transition kinetics is a very interesting subject at high temperature and pressure conditions. By the aid of dynamic diamond anvil cell and XFEL techniques, we will discuss a new discovery of a metastable phase at room temperature which exists in the stable ice VI phase regime and report the hidden multiple crystallization pathways of H2O via the new metastable ice phase and metastable ice VII phase.
Michael Wadas
California Institute of Technology
The mixing induced by a shock wave passing through a fluid interface can stimulate the ejection of high...
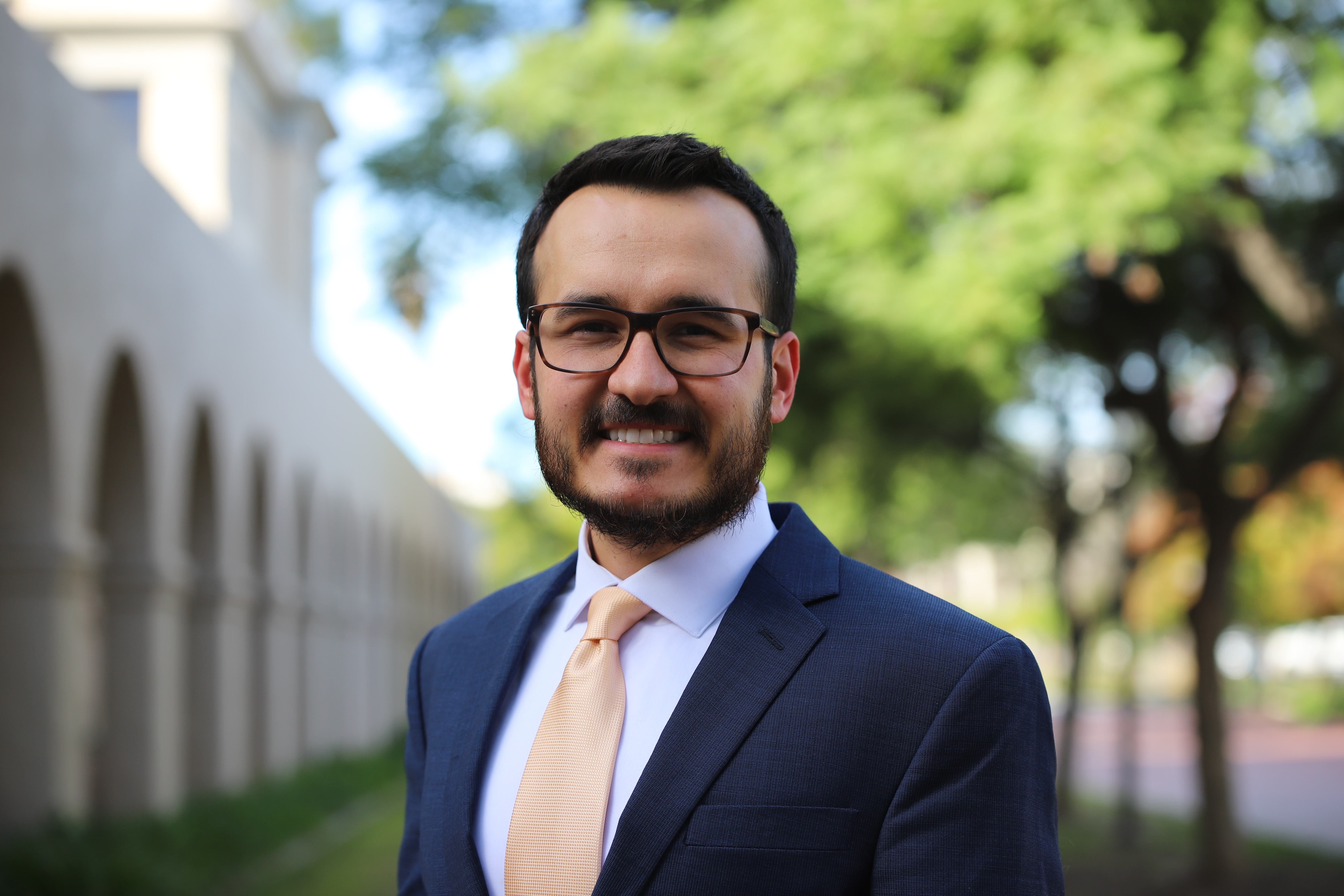
Michael Wadas
California Institute of Technology
The mixing induced by a shock wave passing through a fluid interface can stimulate the ejection of high-velocity projectiles of one fluid into the other, which severely disrupt implosion symmetry in inertial confinement fusion (ICF) and transport stellar core elements during supernovae. Recent improvements in experimental diagnostics and numerical simulations reveal that such projectiles share key characteristics with classical fluid vortex rings, thus enabling a path to understand their dynamics. Our objective is to isolate the ejection of vortex rings from shocked interfaces and determine their scaling through numerical simulations and physical experiments. We find that the strength of the rings expectedly scales with the intensity of density and pressure gradients but saturates beyond a critical protrusion size, enabling an a priori prediction of the energy transported by vortex rings in ICF and supernovae.
Vortex dynamics may have also shaped the environment surrounding the progenitor of Supernova 1987A, which consists of evenly spaced gaseous clumps immersed in an equatorial ring. Our analysis suggests that the ring could have formed an unstable vortex dipole after acquiring vorticity from the progenitor wind, with a dominant wavenumber remarkably consistent with the number of observed clumps. Recent observations by the James Webb Space Telescope further confirm the plausibility that the present vortex instability mechanism induced clump formation.
Krista Soderlund
University of Texas
Planetary magnetic fields exhibit remarkable variations that reveal clues to their deep interio...
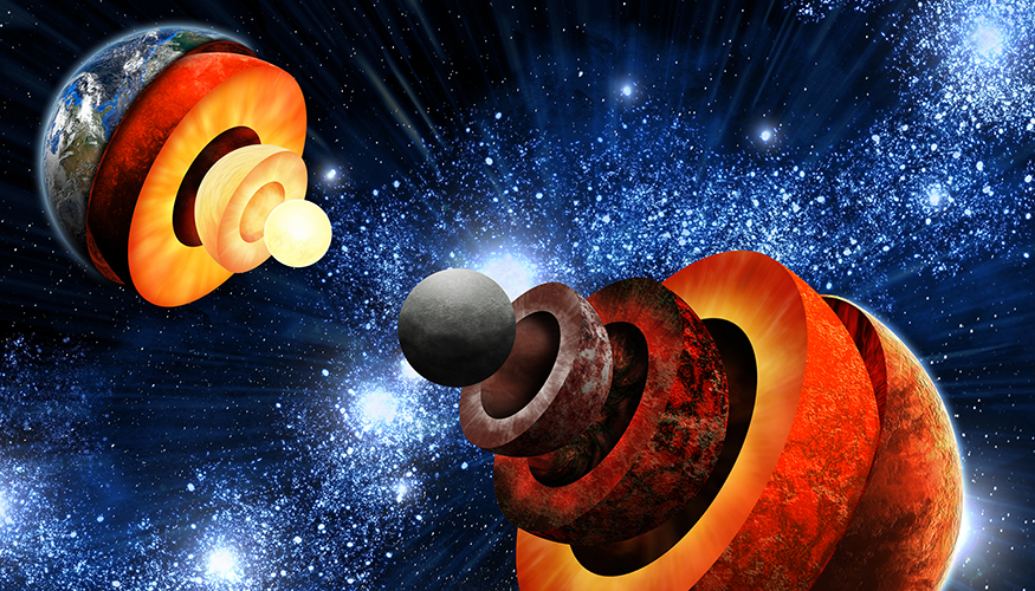
Krista Soderlund
University of Texas
Planetary magnetic fields exhibit remarkable variations that reveal clues to their deep interiors and internal histories. The Voyager 2 flybys, nearly 40 years ago, discovered that Uranus and Neptune have multipolar (i.e., non-dipole dominated) surface magnetic fields with comparable intensities, no clear symmetries along any axis, and unknown temporal variations. In contrast, Saturn and Mercury have strikingly axisymmetric fields and slow secular variation, while Jupiter and Earth have axial-dipole-dominated fields and more rapid secular variation. These observations lead to fundamental questions about what processes control the morphology, intensity, and temporal evolution of planetary magnetic fields. Despite general consensus that Uranus' and Neptune's magnetic fields arise from convection of electrically conducting fluids in their interiors, the mechanisms behind their asymmetric nature remain elusive. To address this gap, we present new results from numerical dynamo models integrating a background density profile that encompasses both interior and deep atmospheric layers, thereby incorporating critical coupling effects between these regions and consistently producing multipolar magnetic fields. Looking ahead, I will conclude by proposing future directions for missions, modelling, experiments, and theory necessary to answer outstanding questions about the dynamos of giant planets, both within our solar system and beyond.
Dominik Kraus
Helmholtz-Zentrum Dresden Rossendorf
The interiors of planets and stars exhibit extreme conditions: High temperatures ...
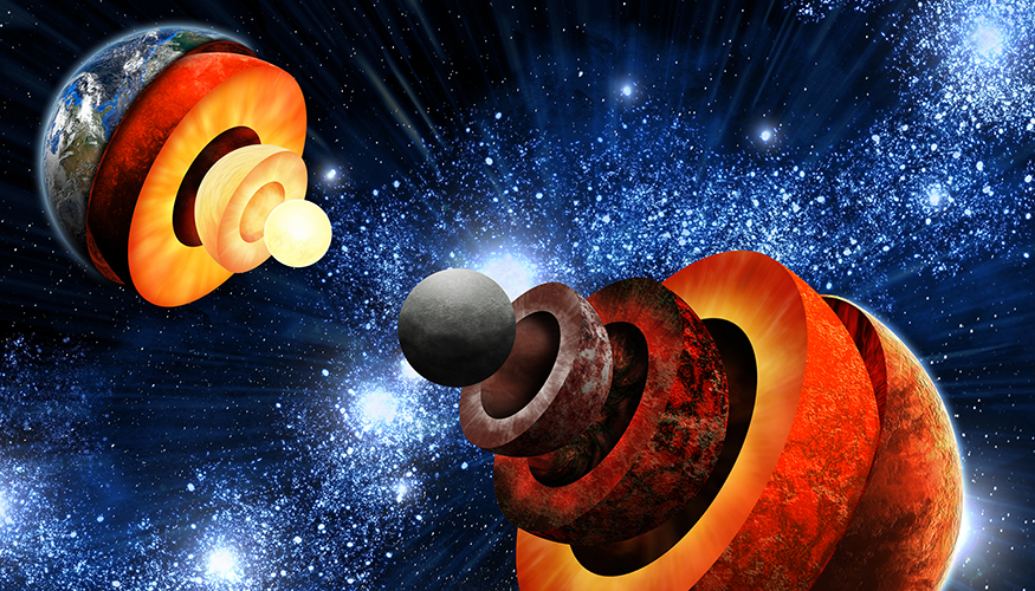
Dominik Kraus
Helmholtz-Zentrum Dresden Rossendorf
The interiors of planets and stars exhibit extreme conditions: High temperatures and enormous pressures create environments which are not fully understood and hard to encompass for state-of-the-art physics models. Applying the largest and most brilliant laser light sources, it is now possible to investigate such conditions in the laboratory. Recent efforts provide seminal insights into chemistry and phase transitions occurring deep inside giant planets and elucidate the electronic structure of elements in the interiors of brown dwarfs and stars. At the same time, highly interesting new materials may be formed via these conditions. Finally, creating stellar interior states in the laboratory may also allow to harvest nuclear fusion, the energy source of the stars, on Earth. I will present a showcase of recent experiments investigating these topics and provide an outlook for future developments, including prospects of the ambitious program "Fusion 2040" initiated very recently by the German government and its potential impact on high energy density science activities in Germany.
Sébastien Thevenin
CEA/DAM
University Paris-Saclay
In this work, we consider the problem of inferring the initial conditions of...
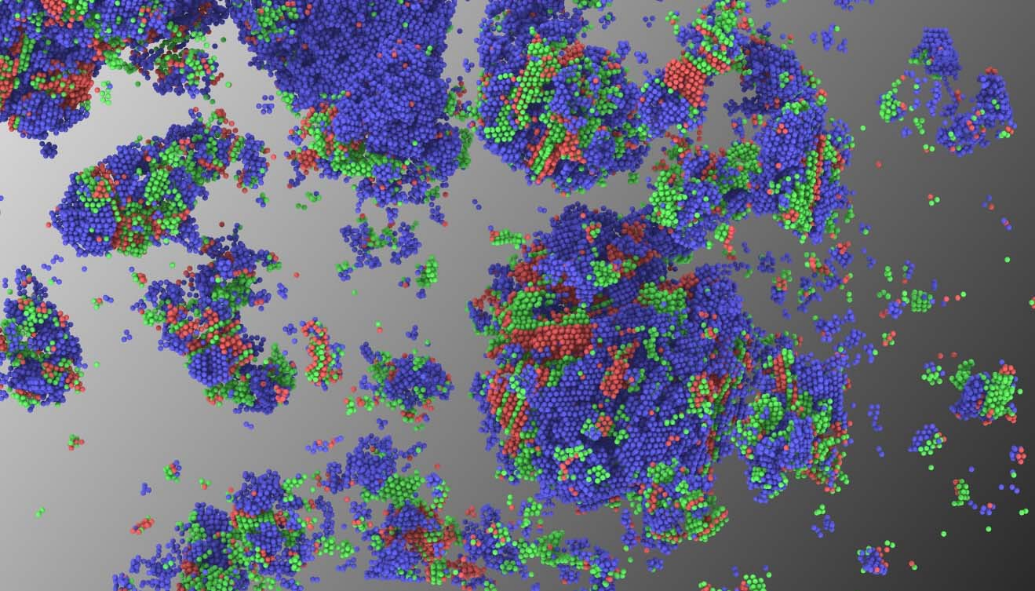
Sébastien Thevenin
CEA/DAM
University Paris-Saclay
In this work, we consider the problem of inferring the initial conditions of a Rayleigh-Taylor mixing zone by measuring the 0D turbulent quantities at an undetermined time. To this aim, we generate a large dataset of direct numerical simulations (DNS) within the framework of small-density contrast miscible fluids and where the initial interface deformations are determined by an annular spectrum parameterized by four non-dimensional numbers. In order to study the sensitivity of 0D turbulent quantities to the initial interface perturbation distributions, we build a surrogate model for the simulations using a physics-informed neural network (PINN). This allows to compute the Sobol indices for the turbulent quantities, disentangling the effects of the initial parameters during the mixing layer growth. Within a Bayesian framework, we use a Markov chain Monte-Carlo method to determine the posterior distributions of initial conditions given various state variables. This sheds light on the inertial or diffusive trajectories along with how the initial conditions are progressively forgotten during transition to turbulence. Besides, it selects which turbulent quantities are more suitable to predict the dynamics of Rayleigh-Taylor mixing zones as keeping better the memory of the flow. By inferring the initial conditions and forward propagating its maximum a posteriori (MAP), we then propose a strategy to model the Rayleigh-Taylor transition to turbulence.
Graeme Sutcliffe
Lawrence Livermore National Laboratory
The Biermann battery is thought to be the mechanism whic...
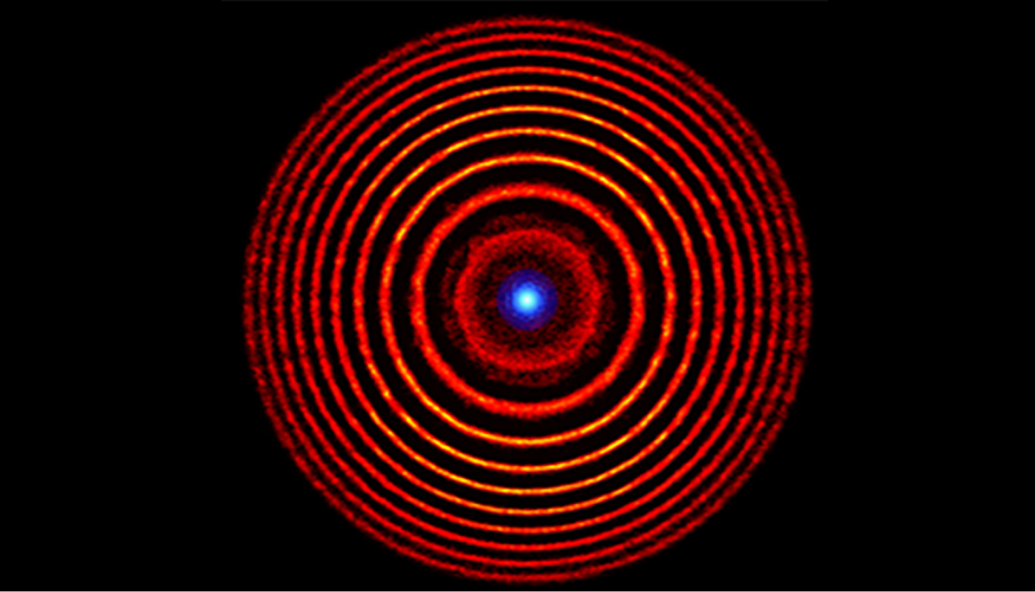
Graeme Sutcliffe
Lawrence Livermore National Laboratory
The Biermann battery is thought to be the mechanism which generates the seed magnetic field in interstellar plasmas after which turbulent dynamo must be invoked to explain how seed fields (~10-20 G) are amplified up to the observed levels (~10-6 G). The Weibel instability is another candidate for the generation of seed fields: PIC simulations suggest that Weibel-generated fields might scale much more favorably for the long astrophysical length scales (𝐵∝𝐿0) than the Biermann-generated fields (𝐵∝𝐿-1). This possibility, however, requires a mechanism to explain how the Weibel fields might grow to larger length scales. Existing theory and simulations describing various details of Weibel filament mergers provide models that can be compared to data: a model agnostic to the magnetic-reconnection microphysics suggests that the long-term evolution of filaments progresses like 𝜆∝𝑡2, while a model invoking magnetic reconnection as the limiting factor in filament mergers suggests that filament wavelengths should follow 𝜆∝𝑡1/2. Distinguishing these models requires linearly saturated ion Weibel filaments, which were demonstrated in an array of prior experiments at OMEGA. One previous study made time-resolved measurements of CH-interpenetrating ion Weibel filaments and found a reasonable fit to the model but did not cover later times where the largest difference between models exists. The experimental campaign described in this talk seeks to investigate this question by pushing measurements to later times and testing the dependence of the merger rate on Lundquist number to see if magnetic reconnection is indeed the limiting physical mechanism in nonlinear Weibel filament evolution.
Dr. Steeve Gréaux
Geodynamics Research Center
Ehime University
Laboratory studies of the elastic wave velocities in minerals under high pressure ...
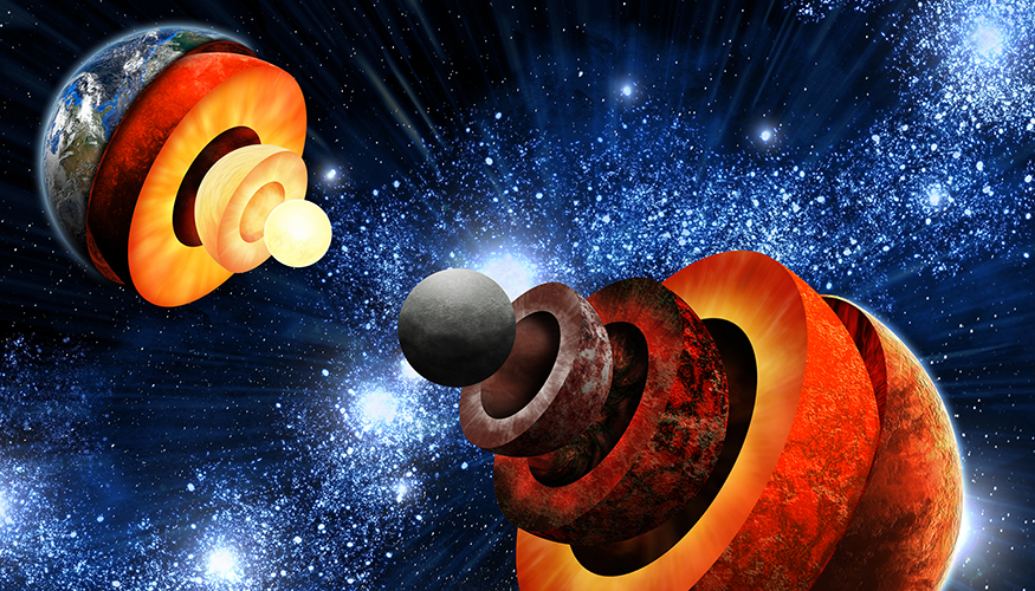
Dr. Steeve Gréaux
Geodynamics Research Center
Ehime University
Laboratory studies of the elastic wave velocities in minerals under high pressure play a major role in enabling scientists to interpret seismic observations in term of composition and structure of the Earth’s deep mantle. While such studies show that the origin of positive seismic discontinuities are mostly attributed to phase transitions, temperature variations, and mineral composition of mantle rocks, the origin of anomalous zones—with low seismic velocities, widespread at multiple depths across the globe—remain much less understood. The mantle transition zone (MTZ) appears to be a very complex layer with seismic velocity increments at the main discontinuities (410’ and 660’) whereas velocities decrements, in addition to high attenuation, are observed in adjacent areas such as between 350-410 km depths atop 410’ and beneath 660.’ The MTZ marks the boundary between the upper and lower mantle and therefore plays a fundamental role in regulating mantle circulation, hence understanding the nature (e.g. mineral composition, presence/absence of melts, etc.) of seismological observations is essential to understand the material cycle of impurities such as water in the Earth's interior.
Several hypotheses have been proposed to explain the observed seismic anomalies, among them, phase transitions associated with mantle temperature variations, the effect of chemistry, and mineralogy or the presence of partial. Here, I will present laboratory measurements of elastic wave velocities by ultrasonic interferometry combined with synchrotron x-ray techniques and the multianvil apparatus. I will show how these techniques are readily used to measure P- and S-wave velocities of mantle minerals and rock aggregates under simultaneous high-pressure and high-temperature, and how those data can be used to constrain the MTZ composition across its major seismic discontinuities. Then, I will show some recent advances for these techniques that allow for investigating the effect of partial melting on the elastic wave velocities of mantle rock aggregates and discuss how those data can be used to investigate anomalous seismic zones in the MTZ.
Dana Dattelbaum
M Division
Los Alamos National Laboratory
Shock-driven reactive flow is relevant to many fields and applicati...
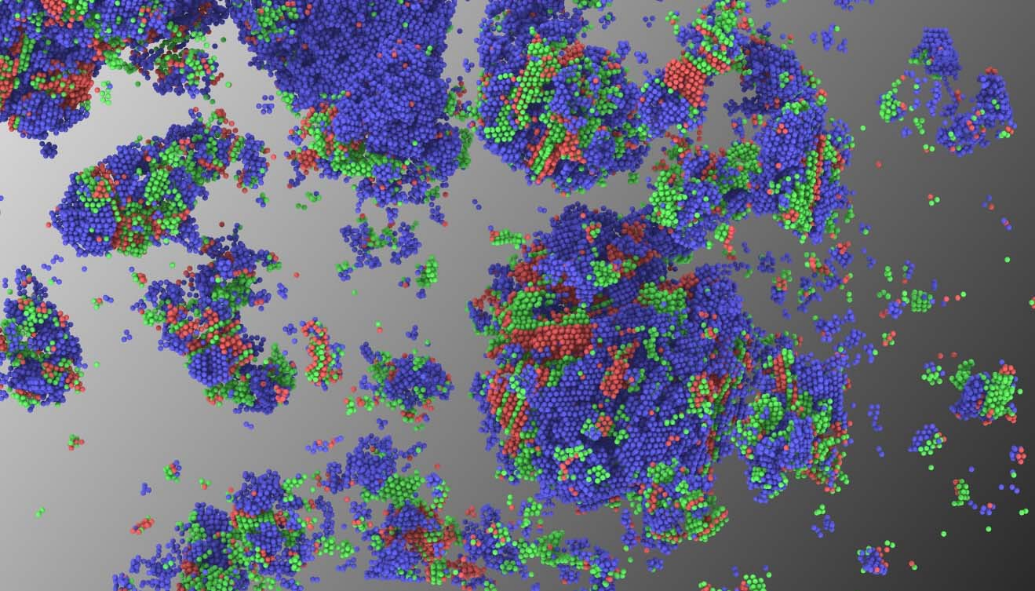
Dana Dattelbaum
M Division
Los Alamos National Laboratory
Shock-driven reactive flow is relevant to many fields and applications related to “extreme condition science,” from detonation physics to shock responses of organic materials and polymers, to reactions of simple molecules relevant to planetary and astrophysics. In this seminar, a review of some of the historical approaches to studying shock-driven chemical reactions will be presented, and recent examples of data from plate impact experiments will be discussed to illustrate what information can be gleaned from measurements of reactive wave profiles. The combination of high brilliance X-rays with dynamic drivers has led to unprecedented insights into shock-driven reactions, and examples will be presented from SLAC-LCLS and APS-DCS facilities. The field of shock-driven chemistry remains a rich field, and new penetrating diagnostics will enable a better understanding of reaction species, their evolution in time, and their dependencies on thermodynamic pathways and states.
Oleg Schilling
Lawrence Livermore National Laboratory
Accurate modeling of molecular (or atomic) transport of mass, momentum, an...
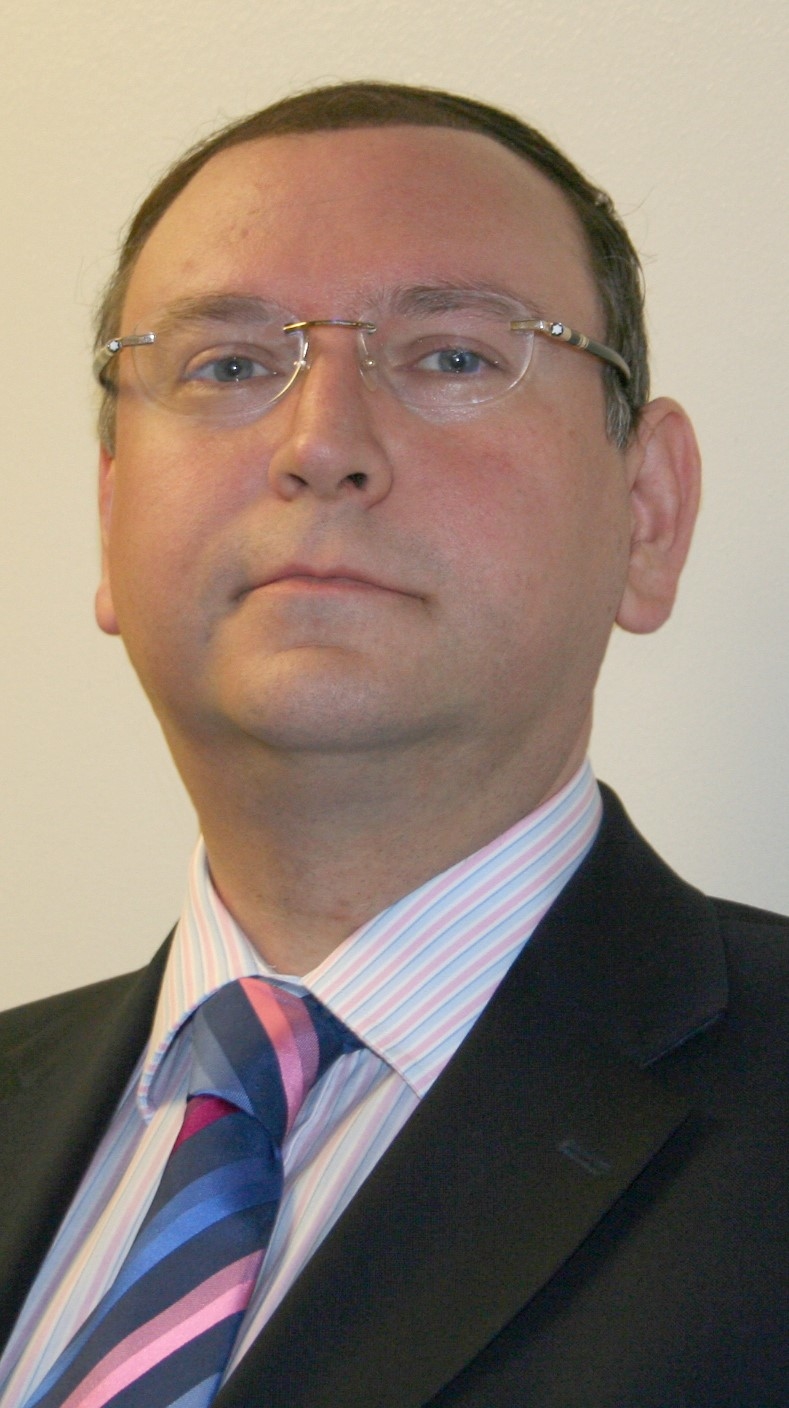
Oleg Schilling
Lawrence Livermore National Laboratory
Accurate modeling of molecular (or atomic) transport of mass, momentum, and thermal energy in single- and multicomponent species mixtures continues to emerge as an important area in the simulation of high-energy-density plasmas and warm dense matter. Much of this is motivated by continuing advances in inertial confinement fusion and laboratory astrophysics, as well as by open questions in both broad subject areas. Many different models have been developed since the pioneering work by Chapman, Cowling, and Enskog over a century ago, and the advent of large-scale molecular dynamics simulations has allowed many assumptions and approximations needed in analytical modeling to be relaxed, resulting in models applicable over a wider range of conditions. This presentation reviews the essential plasma physics required to compute many transport coefficient models, derived both analytically and informed using molecular dynamics, up to the present state-of-the-art. These models are implemented in a standalone Jupyter/Python package, allowing a systematic examination of their predictions over a wide range of conditions. The structure and capabilities of this package are briefly described. The package is then used to calculate the ionic binary mass diffusivities, viscosities, and thermal conductivities for a variety of mixtures with increasing nuclear charge asymmetry (D-T, D-C, D-Al, and D-Au). Similarities and differences between the model predictions are discussed. Some of the current outstanding challenges in further development and application of the models are briefly highlighted. Finally, future work using the package is summarized.
Hyeon Park
Ulsan National Institute of Science and Technology, Korea
Historically, fusion research started with the study of the energy source of stars ...
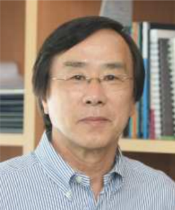
Hyeon Park
Ulsan National Institute of Science and Technology, Korea
Historically, fusion research started with the study of the energy source of stars and the sun. Following successful uncontrolled fusion experiments, the effort for developing controlled fusion energy has been accelerated. The accumulated performance and confinement scaling data of the magnetic fusion plasmas for nearly half a century enabled construction of ITER to challenge ignition in magnetic fusion. The critical path is the sustainment of a stable high-density plasmas with Ti>10keV. This talk critically reviews research results of magnetically confined fusion plasmas for half a century. In particular, improved confinement regimes with edge transport barrier (ETB) (i.e., H-mode) and/or internal transport barrier (ITB) (i.e., super-shot, super-H-mode, etc.) are attributed to efficient core heating of ions and electrons in different magnetic configurations in contrast to the turbulence suppression physics models developed for ITB and ETB. Control of MHD instabilities of the magnetically confinement plasma such as Sawtooth, Tearing Mode (TM), and Edge Localized Mode (ELM) is critical for steady state operation and possible remedies are suggested. A most probable ignition device equipped with control tools of confinement, stability, and efficient heat exhaust is suggested. The main objective of the ignition device is to test the threshold electron heating for Ti>10keV through a-power up to ~50 MW (~200 MW of fusion power) in 1/4 of plasma volume of ITER for is explored.
Professor Allen Boozer
Columbia University
The advection-diffusion equation was used by Aref in 1984 to explain the rapidity of mixing in fluids, such a...
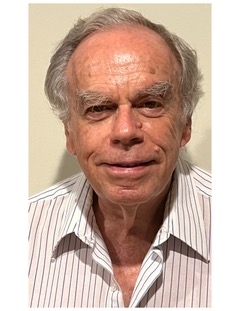
Professor Allen Boozer
Columbia University
The advection-diffusion equation was used by Aref in 1984 to explain the rapidity of mixing in fluids, such as temperature equilibration in a room. Although equilibration cannot occur without diffusion, the equilibration occurs on timescale only an order magnitude longer than the timescale of the flow—essentially independent of how small the diffusion may be. Chaos in the flow is essential, an exponential increase in time of the separation of neighboring streamlines. Essentially, all natural flows are chaotic—including smooth laminar flows. It is not generally appreciated that Faraday’s Law and the Fokker-Planck equation are of the advection-diffusion form. For chaos to affect the evolution of a magnetic field, three-dimensional space is required; two-dimensional models exclude chaos. A near-ideal evolution of a magnetic field has three aspects: (1) Chaos allows large-scale breaking of field-line connections on a timescale approximately an order of magnitude longer than the ideal evolution timescale even though a non-ideal effect such as resistivity is required. (2) The magnetic energy released by the breaking of connections is not directly dissipated but goes into Alfvén waves. The Alfvén waves are resistively damped when the current density reaches a value inversely proportional to the resistivity. This occurs quickly after large-scale connection breaking. (3) Magnetic helicity can be inserted into a volume by boundary conditions, but its resistive dissipation is extremely slow—on the global resistive timescale.
The Vlasov operator is the advective part of the Fokker-Planck equation and the collision operator the diffusive part. Without collisions there can be no change in the entropy per unit volume, but chaos in the particle trajectories causes velocity space gradients to steepen exponentially in time. The implication is that entropy can be created at a rate that is essentially independent of the collision frequency. Unless the spatial scale of the chaos is very short, the entropy increase, and the associated transport are too great to be consistent with fusion power. But microturbulent driven chaos on the ion gyroradius scale gives gyroBohm diffusion. GyroBohm diffusion is independent of the collision frequency but is consistent with fusion power. Forty years ago, the absence of a continuous symmetry was thought to be a fatal flaw of the application of stellarators to fusion, but W7-X demonstrated that large-scale chaos can be eliminated. Stellarators now appear to offer the minimum time and risk path to fusion power—largely because stellarators offer the most complete external control of the plasma.
Charles Arrowsmith
Department of Physics
University of Oxford
I will present results of an experiment performed at CERN aimed at creating a labor...
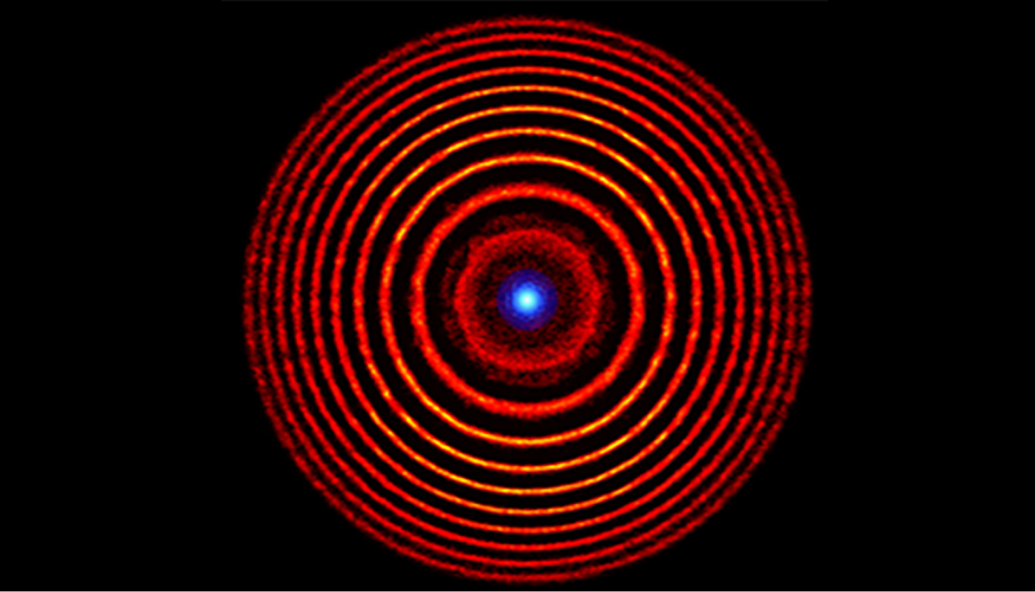
Charles Arrowsmith
Department of Physics
University of Oxford
I will present results of an experiment performed at CERN aimed at creating a laboratory analogue of ultra-relativistic astrophysical pair jets propagating through intergalactic tenuous plasma. More than 1,013 electron-positron pairs are produced by irradiating a solid target with 440 GeV protons from the Super Proton Synchrotron, and the produced pair beam exceeds multiple Debye screening volumes. Linear kinetic plasma theory predicts that the pair beams should be unstable to filamentation (Weibel-type) beam-plasma instabilities as the beams propagate through a 1-m length plasma. However, in our experiment we observed the pair beams to be remarkably stable. When non-idealized beam conditions are assumed in theoretical calculations, such as the inclusion of a small beam divergence (e.g. transverse beam momentum a few percent of the longitudinal momentum), the instability is dramatically suppressed. The strong suppression is also observed in 3D particle-in-cell simulations modelling the beam-plasma interaction. Implications are wide-reaching, as beam-plasma instabilities are commonly invoked as a means of energy dissipation and synchrotron emission via self-generation of magnetic fields in astrophysical pair jets (for instance in models of Gamma-ray bursts). The maximum instability growth rate inferred from the experiment is scaled to an example case: blazar-induced pair jets propagating through cosmic voids, with the conclusion that the growth rate of the instability is too slow to significantly disrupt the pairs before they Compton scatter with cosmic microwave background radiation.
Bin Wang
University of Oklahoma
Interfacial charge transfer and excitation play a valuable role in breaking and forming chemical bon...
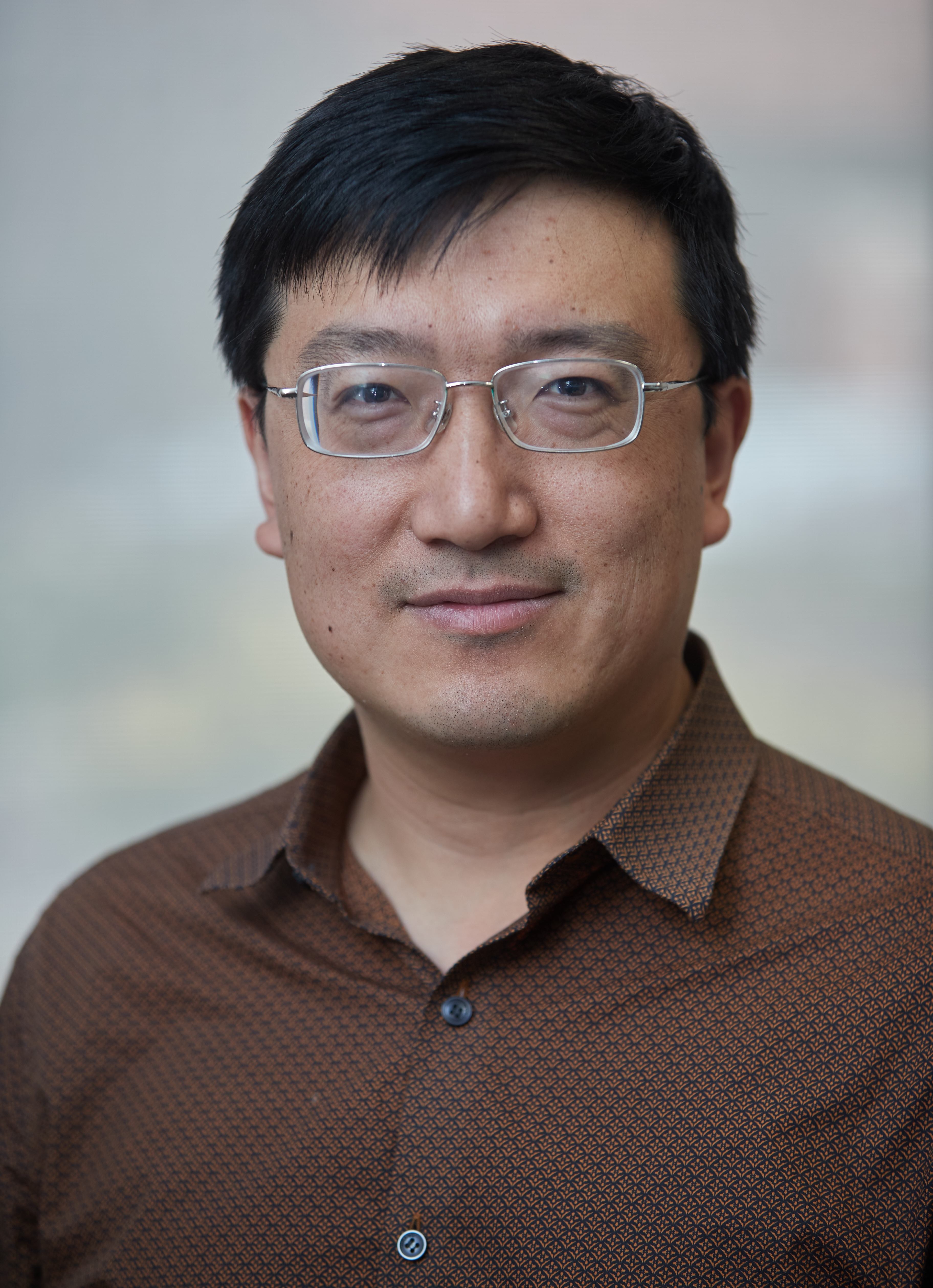
Bin Wang
University of Oklahoma
Interfacial charge transfer and excitation play a valuable role in breaking and forming chemical bonds in materials and molecules. Here I report case studies where plasmonic “hot” electrons directly participate in the bond dynamics for more efficient and selective structural and chemical transformation. I will focus on electronic excitation in plasmonic materials. Through electronic structure calculations, we show that the hot electrons can be injected into metal oxides and drive the structural phase transition by coupling with specific phonon modes. In plasmonic catalysis, we find that the non-equilibrium energized electrons, generated through direct interfacial charge transfer, may be responsible for the observed rate enhancement and/or selectivity change in several reactions, based on which we propose that the selective population of molecular unoccupied orbitals is critical for the catalytic reactions.
Nathaniel Shaffer
Laboratory for Laser Energetics
University of Rochester
Thermal conduction is a key component of the energy...
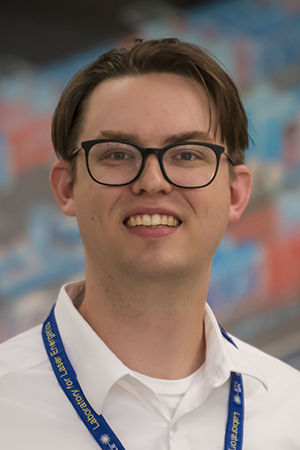
Nathaniel Shaffer
Laboratory for Laser Energetics
University of Rochester
Thermal conduction is a key component of the energy balance and transport of laser-produced plasmas. The baseline thermal conduction model used in radiation-hydrodynamics modeling of HED experiments is the Spitzer-Harm model, which is based on a Maxwell-Boltzmann local equilibrium. This is a fragile assumption that is easily violated. One way is when the electrons couple to radiation fields. The most prominent such mechanism in laser plasmas is inverse bremsstrahlung (IB) absorption. For intense laser light, IB is a kinetic process that drives electrons out of a Maxwell-Boltzmann distribution, with compounding effects on transport. Backed by kinetic Vlasov-Fokker-Planck simulations, I will show how IB absorption leads to intensity-dependent transport coefficients and provide a practical correction to the Spitzer-Harm model for use in radiation-hydrodynamics simulations. Time-permitting, I will give some attention to intensity effects on non-local conduction as well.
Dr. Hui Chen
Lawrence Livermore National Laboratory
The study of relativistic electron–positron pair plasmas is both of fundamental physics interest and...
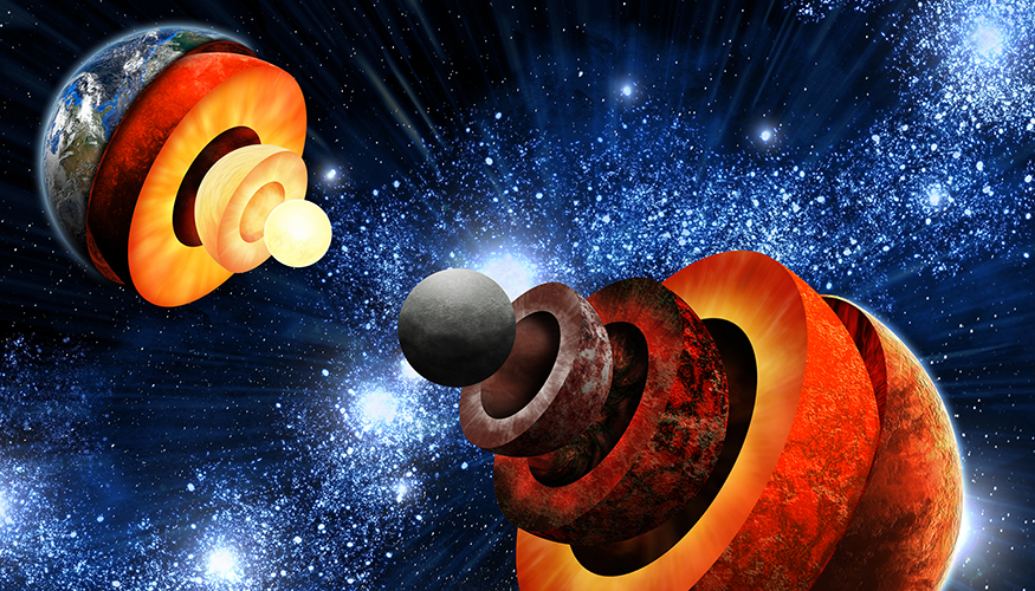
Dr. Hui Chen
Lawrence Livermore National Laboratory
The study of relativistic electron–positron pair plasmas is both of fundamental physics interest and important to understand the processes that shape the magnetic field dynamics, particle acceleration, and radiation emission in high-energy astrophysical environments. Although it is highly desirable to study relativistic pair plasmas in the laboratory, their generation and control constitutes a critical challenge. Significant experimental and theoretical progress has been made over recent years to explore the use of intense lasers to produce dense relativistic pair plasma in the laboratory and study the basic collective plasma processes associated with these systems. Important challenges remain in terms of improving the number of pairs, system size, and control over the charge neutrality required to establish laboratory platforms that can expand our understanding of relativistic pair plasma and help validate underlying models in conditions relevant to high-energy astrophysical phenomena. We will highlight recent progress in this field, discuss the main challenges, and explore the exciting prospects for studying relativistic pair plasmas and astrophysics relevant instabilities in the laboratory in the near future.
Blagoje Djordjevic
Lawrence Livermore National Laboratory
Computer models of intense, laser-driven particle acceleration require expensive particle-in-c...
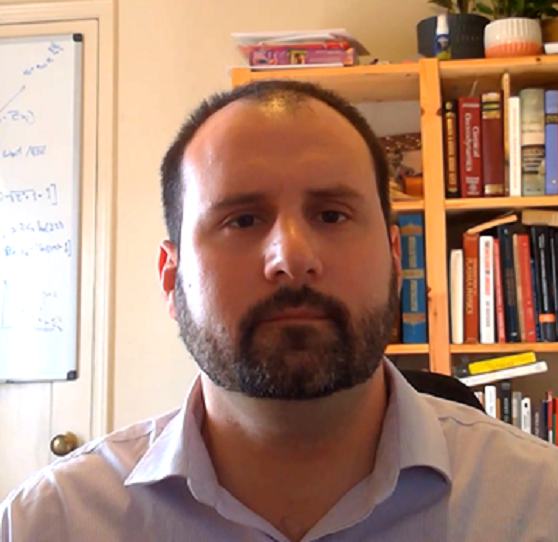
Blagoje Djordjevic
Lawrence Livermore National Laboratory
Computer models of intense, laser-driven particle acceleration require expensive particle-in-cell (PIC) simulations that may struggle to capture all the multi-scale, multi-dimensional physics involved at reasonable costs. As a short-pulse laser impinges on a solid target, the system evolves under a variety of conditions, hydrodynamic and kinetic, in ways that complicate efforts to simply model and understand the physics underway, let alone optimize the particle beams and radiation generated. Explored here is a multi-fidelity approach to ameliorate this deficiency that can incorporate physical trends and phenomena at different levels. As the base framework for this study, an ensemble of approximately 10,000 1D PIC simulations was generated to buttress separate ensembles of hundreds of higher fidelity 1D and 2D simulations. Using transfer learning with deep neural networks, one can reproduce the fidelity of more complex physics at a much smaller cost. The networks trained in this fashion can in turn act as surrogate models for the simulations themselves, allowing for quick and efficient exploration of the parameter space of interest. These surrogate models are useful for exploring more complex schemes, such as pulse shaping, material studies, different acceleration mechanisms, etc. This work was also done in synergy with experimental efforts to develop and optimize high-repetition-rate laser systems for particle and light source applications.
View seminar presentation slides
David Bruhwiler
RadiaSoft LLC
The ultrafast laser community has called out for broadly available modeling software that self-consistently captures the r...
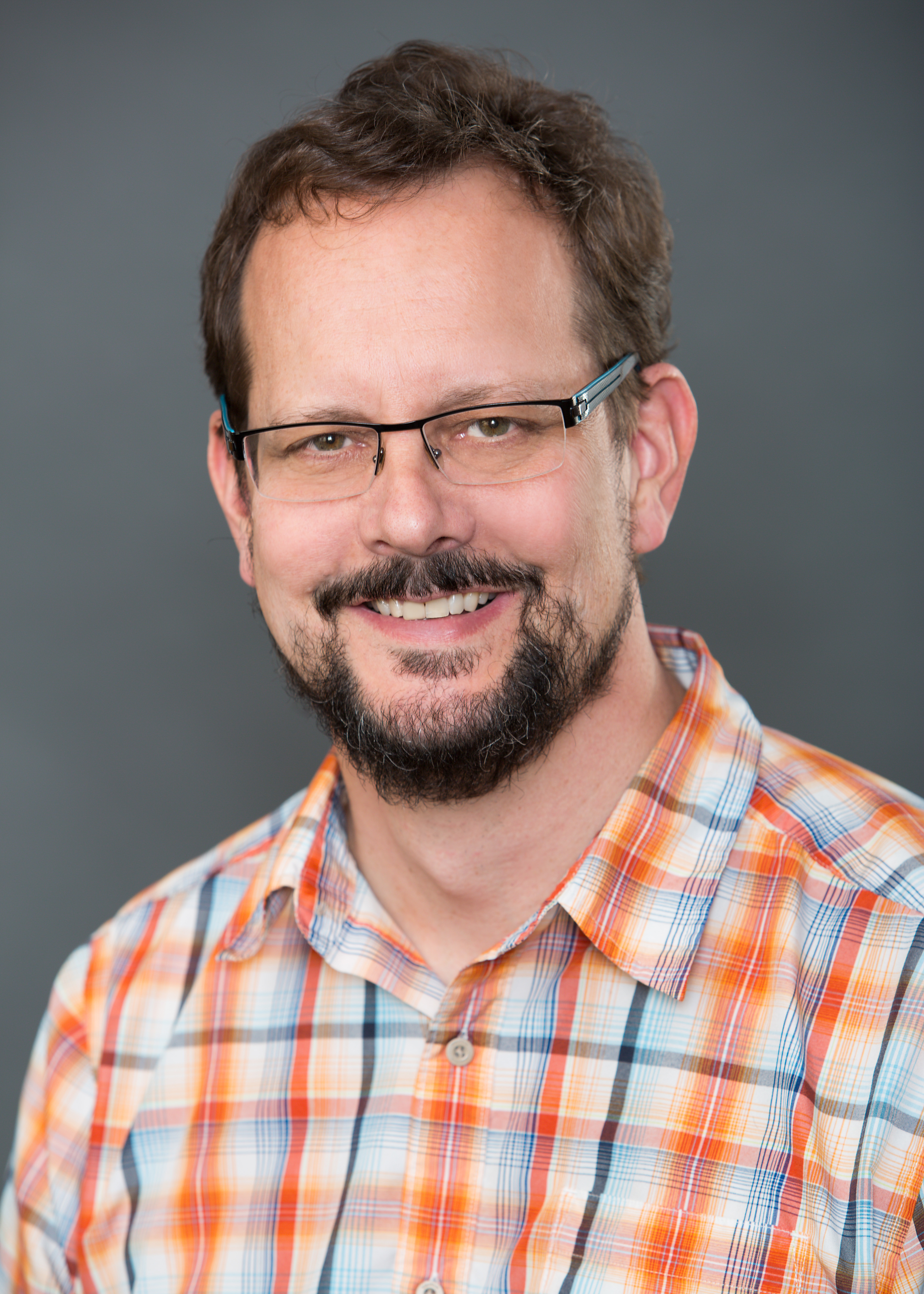
David Bruhwiler
RadiaSoft LLC
The ultrafast laser community has called out for broadly available modeling software that self-consistently captures the required physics of gain, thermal loading and lensing, spectral shaping, and other effects required to simulate and optimize high-intensity lasers (100 TW to multi-PW) with ultra-short pulse lengths (< 100 fs). We are addressing this need by developing an open-source library. Our operator splitting approach divides each crystal and the laser pulse into slices. Each laser slice is represented by a monochromatic 2D wavefront, with intrinsic bandwidth captured via overlapping slices. The 1D Frantz-Nodvik equation accurately captures amplification on a cell-by-cell basis within a 2D Cartesian mesh. Thermal lensing uses a near-axis expansion. Simulations show good agreement with experimental data, including the redshift during amplification. We will review the Sirepo-Silas app for executing these simulations in your browser and discuss the Sirepo cloud computing framework. Sirepo supports computing workflows from command-line to GUI, including built-in support for NERSC. Sirepo facilitates code coupling, rapid benchmarking, computational reproducibility, and easy collaboration for distributed teams. The Sirepo interface to the FLASH code demonstrates how a responsive, adaptive GUI enables high-performance computing from the browser.
Otto Landen
Lawrence Livermore National Laboratory
The discovery of X-rays by W. Roentgen coincided with the fir...
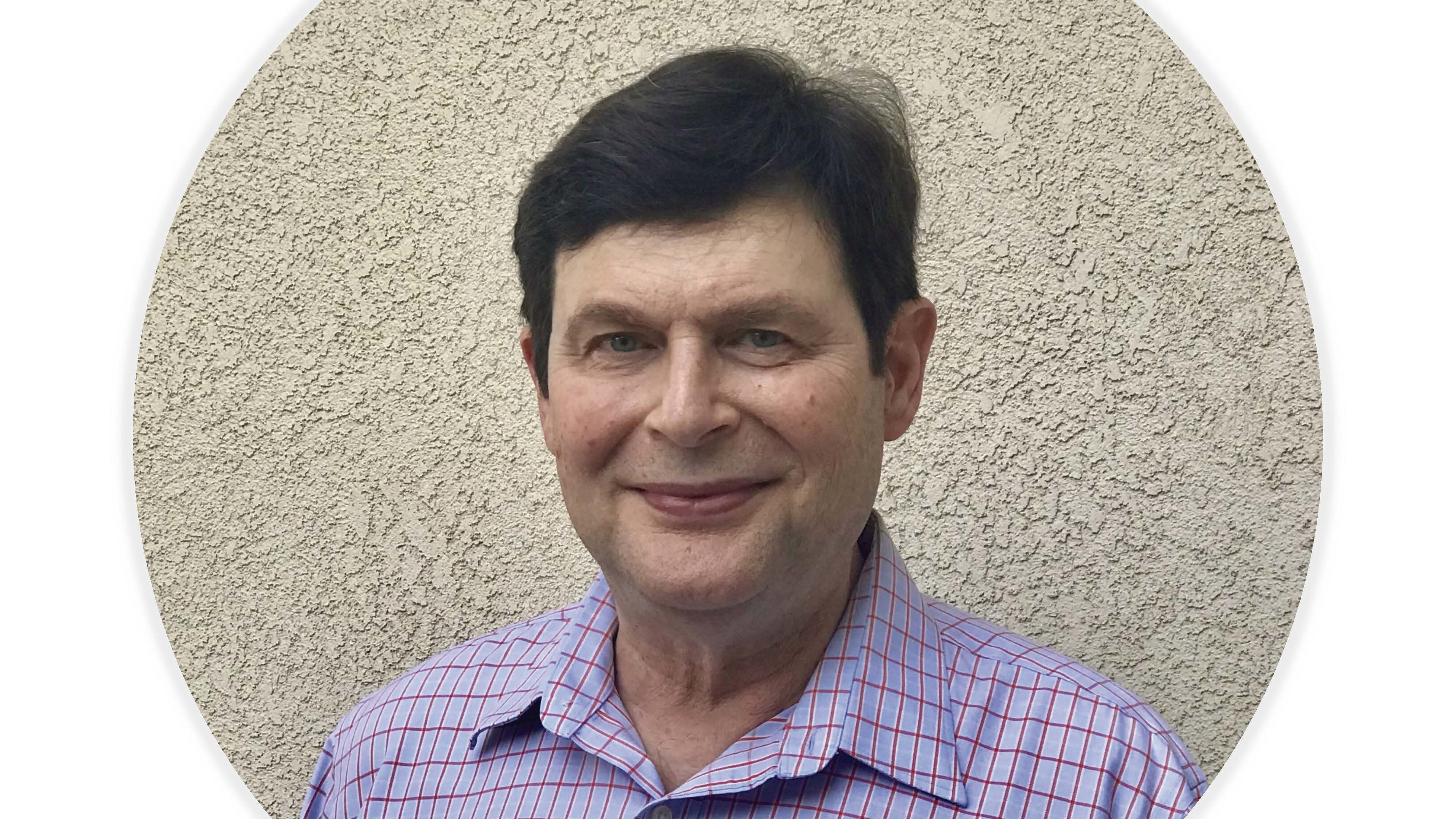
Otto Landen
Lawrence Livermore National Laboratory
The discovery of X-rays by W. Roentgen coincided with the first radiograph of a dense static object. This was followed by demonstrations of spectrally resolved Compton scattering [2] probing bound electron momenta [3] in solids, but the realization that of limited use over the temperature ranges then available in the laboratory [4]. Fast-forward to 1975 onwards, dense 10 eV – 10 keV plasmas created in High Energy Density (HED) and Inertial Confinement Fusion (ICF) facilities are routinely probed by intense laser-produced x-ray sources coupled to gated and streaked detectors [5]. This beckoned the development of increasingly sophisticated transient x-ray radiography [6-9] and spectrally resolved x-ray scattering [10-13]. This talk will review my journey in developing active x-ray probing techniques involving multiple teams, that in hindsight was surprisingly nonlinear in progression. I will summarize the main physics learned and what further impactful developments could lie in the near future, with the caution, paraphrasing E. Teller, that we develop new capabilities precisely because we don’t know what high impact applications and discoveries will be enabled by those capabilities in the future.
Dr. Yuki Abe
Osaka University
The "inverted-corona" fusion scheme has been investigated as an alternative approach to inertial confinement fusion (ICF) ...
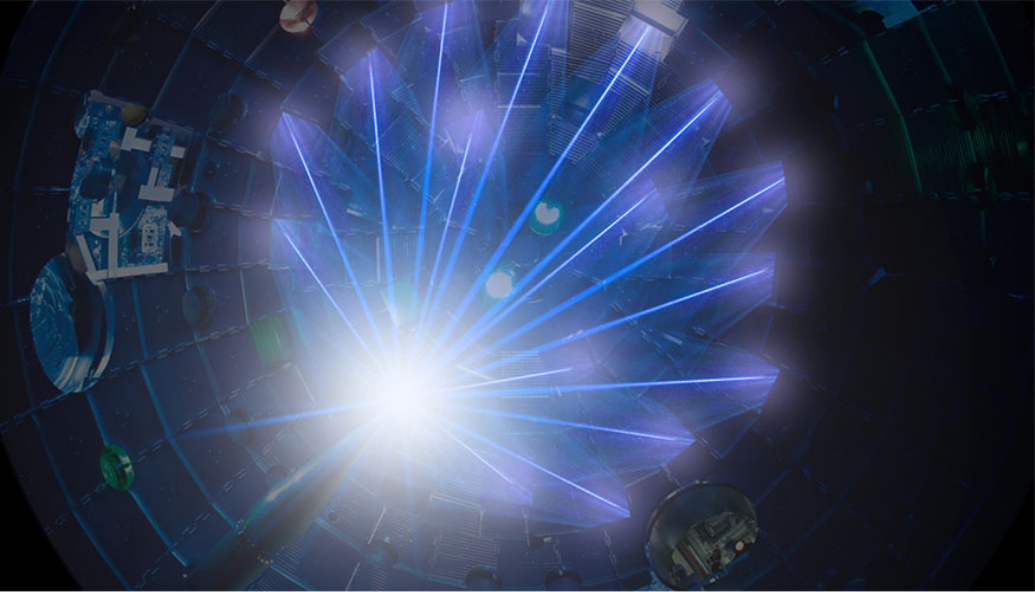
Dr. Yuki Abe
Osaka University
The "inverted-corona" fusion scheme has been investigated as an alternative approach to inertial confinement fusion (ICF) with improved robustness to significant laser-drive asymmetries. The inverted-corona fusion is based on the laser ablation inside a hollow target (e.g., a spherical capsule or cylindrical capillary). The ablative plasma convergence results in the generation of burning plasma core with extremely high temperatures (Ti ~ 10 keV) and modest densities (ni ~ 1e21 /cc) that could be a good point neutron source with diameters of less than 100 um. Such target designs are robust to low-mode drive asymmetries, allowing single-sided laser drive with neutron performance comparable to nearly spherically symmetric drive. Recently, we have demonstrated significant neutron enhancement by an advanced inverted-corona scheme, where lasers with relativistic intensities are used to drive TNSA-induced plasma convergence. Here, we discuss our recent experimental results on the inverted-corona fusion scheme and its potential applications in high energy density physics.
Jack Whaley-Baldwin
Cambridge University
Knowledge of the crystal structure of a solid-state material allows, in principle, for the ...
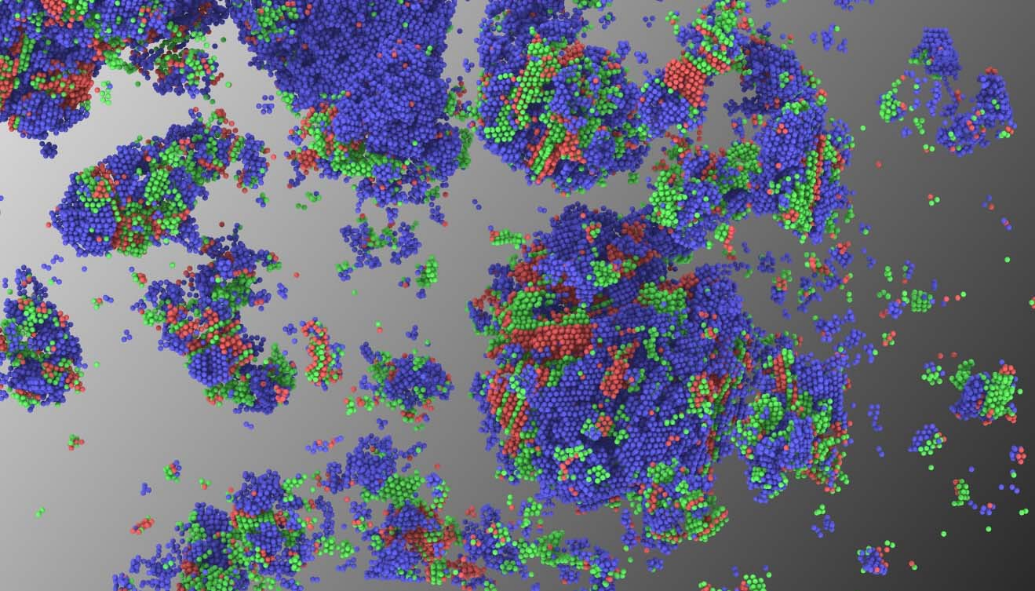
Jack Whaley-Baldwin
Cambridge University
Knowledge of the crystal structure of a solid-state material allows, in principle, for the computation of any property of interest; there exists a plethora of modern materials modeling codes which require only the geometry of a material as input. In many cases, crystal structures are lifted from experimental x-ray diffraction (XRD) results, which can then be fed into a code of choice. However, this procedure fails when there is no experimental data available, perhaps because the conditions of interest are too extreme for reliable XRD, or simply that the experiment has not yet been carried out.
The application of extreme pressure has yielded a plethora of unexpected and counter-intuitive phenomena in materials, such as metals becoming insulators, and even insulators becoming superconductors. Yet, it is precisely this high-pressure regime where experimental data is lacking in many systems, and computational techniques can offer vital insight.
In this talk, I will discuss the use of Random Structure Search (RSS) and Density Functional Theory (DFT) to construct phase diagrams at multi-terapascal (TPa) pressures given no prior information (i.e., ab-initio). I will provide a general overview of prior works on TPa crystal structure prediction, before focusing in detail on the cases of sulfur, potassium, and lithium, where the structural and electronic properties of these elements will be investigated in detail.
Patrick Audebert
Laboratoire pour l’Utilisation des Lasers Intenses, CNRS
Ecole Polytechnique, Université Paris-Saclay
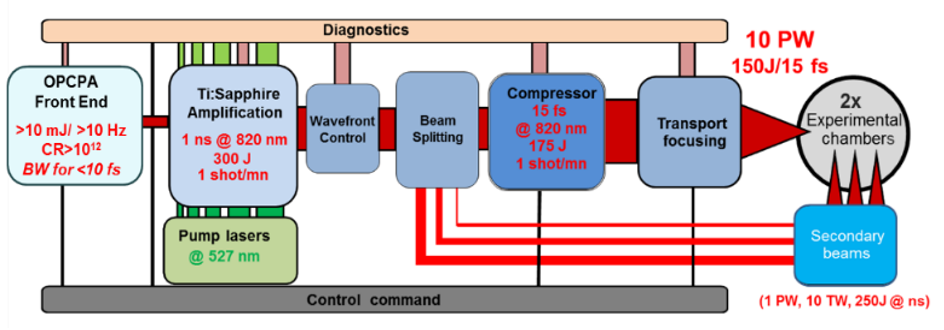
Patrick Audebert
Laboratoire pour l’Utilisation des Lasers Intenses, CNRS
Ecole Polytechnique, Université Paris-Saclay
The Apollon laser, currently under construction at Orme des Merisiers, Saclay, France, aims to be among the first multi-PW facility in the world devoted to the study of high intensity laser matter interaction at unprecedented regimes and peak intensities above 2x1022 W/cm2. The final goal of the Apollon laser is the generation of 10 PW pulses corresponding to an energy of 180 J and 18 fs duration at a repetition rate of 1 shot/minute. The architecture of the Apollon laser is hybrid, combining a high contrast OPCPA based Front End followed by 5 Ti:Sapphire multipass amplifiers capable of reaching up to 300 J before compression. Apollon provides up to four beam lines (10 PW, 1 PW, 10 TW, uncompressed beam), all generated by the same beam after the last amplifier with the possibility to be combined on the target under different geometries and synchronization configurations. The Apollon facility offers two radio-protected experimental areas: 1) The Long Focus Area (LFA) where mostly gas targets and electron acceleration experiments are realized and 2) the Short Focus Area (SFA) where tight focusing (F#2.5) on solid targets and ion acceleration is the principal objective.
In this work we will provide an overview of the construction progress of the facility emphasizing on the recent commissioning of the 1 PW beamline of the system. Details will be provided on the obtained performances regarding the pulse contrast, the focal spot quality as well as the overall operational stability of the laser. In a second part we will present a summary of the results of the different experimental campaigns carried out in both experimental areas on the PW level. The objectives of these experiments includes high energy electron acceleration, electron-positron pair generation, proton acceleration, as well as high harmonics generation. In the last part, we present an overview of the mission profile used for the user community experiments and of the planned commissioning of the 10 PW beamline and the preliminary experiments at the multi-PW level.
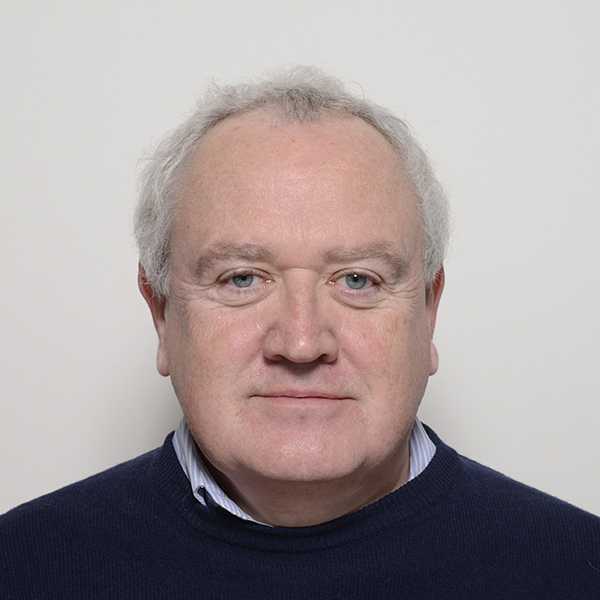
David Hoarty
Atomic Weapons Establishment
Recent measurements at the Sandia National Laboratory of the x-ray transmission of iron plasma have inferred opacities much higher than predicted by theory, which casts doubt on modeling of iron x-ray radiative opacity at conditions close to the solar convective zone-radiative zone boundary. An increased radiative opacity of the solar mixture, in particular iron, is a possible explanation for the disagreement in the position of the solar convection zone-radiative zone boundary as measured by helioseismology and predicted by modelling using the most recent photosphere analysis of the elemental composition. Here we present data from radiation burn-through experiments, which do not support a large increase in the opacity of iron at conditions close to the base of the solar convection zone and provide a constraint on the possible values of both the mean opacity and the opacity in the x-ray range of the Sandia experiments. The data agree with opacity values from current state-of-the-art opacity modelling using the CASSANDRA opacity code.
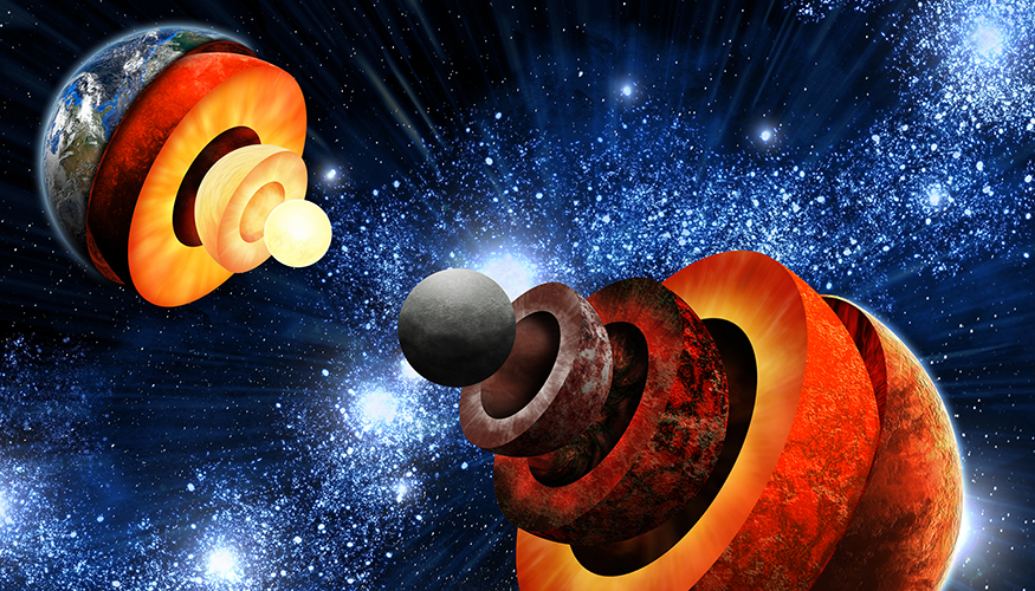
Didier Saumon
Los Alamos National Laboratory
White dwarfs are a class of common stars with uncommon physical properties. Their most unique characteristic is that they consist of about a solar mass of matter confined to a volume as small as the Earth. This results in an unearthly density of ~ 106 g/cm3. White dwarfs present many challenging problems that can only be solved with advanced theories of dense matter, state-of-the-art experimental techniques, and extensive computing efforts.
I will discuss the nature of white dwarfs, the physical conditions they span and a few selected problems that are broadly related to equations of state, transport processes and opacities in the warm dense matter regime. These include inter-diffusion coefficients in the moderately coupled regime, thermal conductivity of H and He in the partially degenerate regime, measurements of the EOS of carbon as well as the ionization and opacity of He.
Ian Ocampo
Princeton University
Laser-driven dyn...
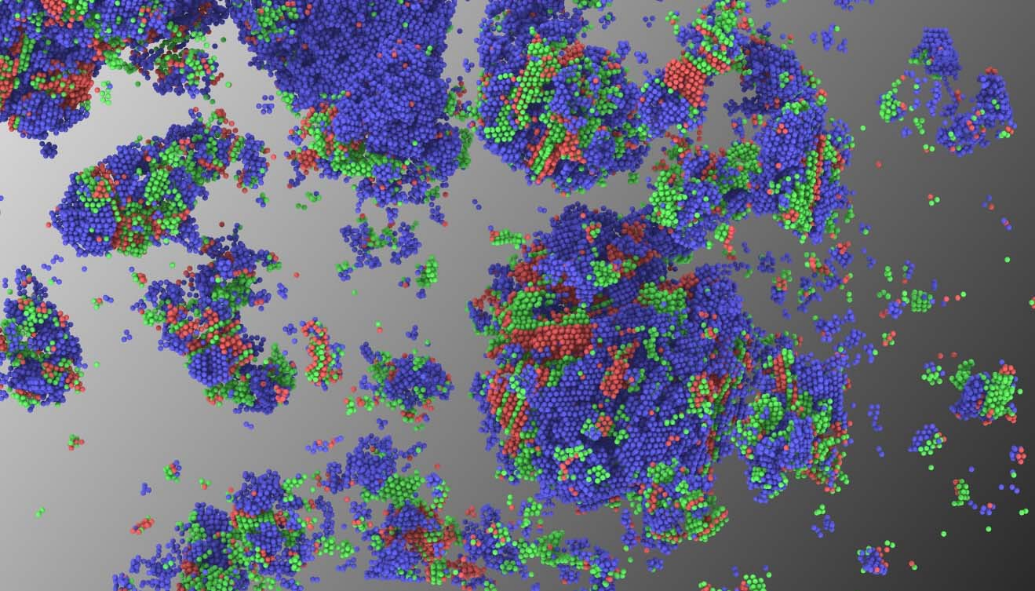
Ian Ocampo
Princeton University
Laser-driven dynamic compression enables the study of planetary materials (e.g., cosmo chemically abundant oxides) at the extreme pressure-temperature (PT) conditions of Earth’s deep interior. When coupled with in situ x-ray diffraction, fundamental properties such as crystal structure and equation of state can be accurately measured which are essential for developing interior structure, dynamics, and evolution models for planets within and outside our solar system. The discovery of over 5500 extrasolar planets has motivated research on matter at even more extreme PT conditions as calculations based on density estimates for these planets suggest PT profiles that greatly exceed those for Earth. As planetary detection techniques and mass and radius measurement precision continue to improve, experimental efforts must accompany these advances to better understand the interiors of these planets which largely control their surface processes, including planet habitability.
In this seminar, I will present new results from two such studies where in situ x-ray diffraction is used to diagnose phase stability and crystal structure of planetary oxides dynamically compressed to multi-megabar pressures. In part one, I will highlight recent results on single crystal TiO2, an abundant crustal mineral and an important analog system for SiO2, shock compressed to ~150 GPa. We observe transformation to a highly coordinated structure predicted to exist in the SiO2 system at pressures greater than 600 GPa, almost twice that at the center of Earth. In part two, I will discuss results on iron oxides (Fe2O3 and Fe3O4) ramp compressed to ~700 GPa. Iron oxides act both as analogs of Mg-silicates as well as markers of mantle redox. Our results provide important insights into exoplanet mineralogy and, when combined with previous works on Fe and FeO, are then used to develop a compositional model for the Earth’s solid inner core.
Dr. Thomas Gomez
University Texas at Austin
H...
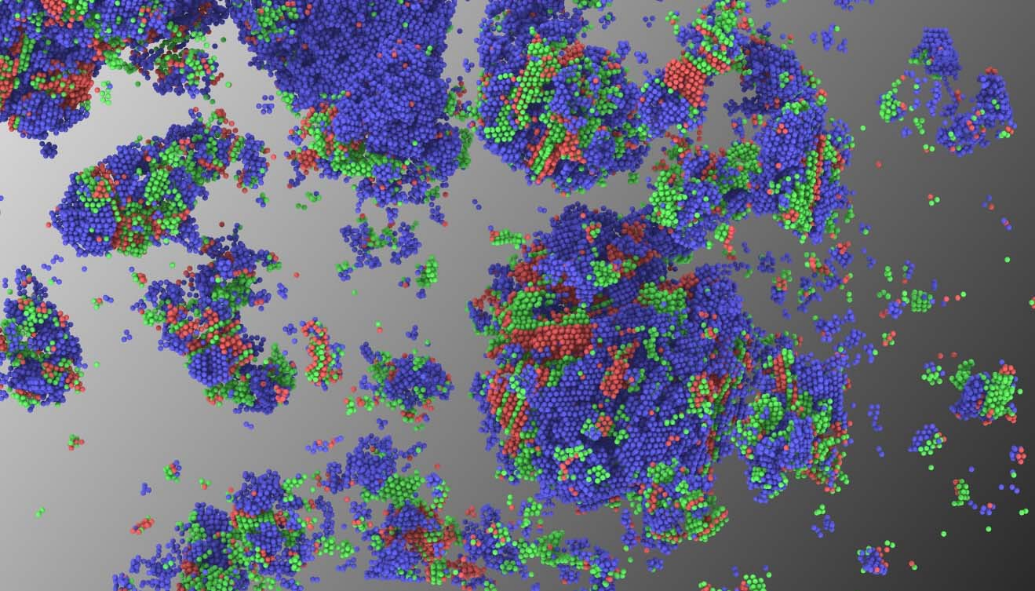
Dr. Thomas Gomez
University Texas at Austin
Hot dense plasmas are found in both laboratory and astrophysical environments. One of the principal ways in which we can understand these plasmas is by analyzing their spectra. Proper analysis requires the most up-to-date models that capture the relevant plasma processes. One of the most direct ways in which the plasma environment affects a spectrum is through the broadening of the lines. Spectral line shapes are important as a diagnostic, helping to determine the electron density of a plasma. Line shapes also affect the opacity of material since broader spectral lines increase the Rosseland mean opacity.
The physics of line shapes are complex because they include many different physical effects. For instance, they include detailed modeling of collisions/scattering between plasma particles and the atomic radiators in that plasma. The bulk properties of the plasma also need to be considered, for instance, the screening of charges. Lastly, appropriate statistics of certain processes need to be accounted for. Unfortunately, the study of spectral line shapes has all but disappeared in the U.S. Recently, however, interest has been renewed and substantial advances in the field are being led at the University of Texas.
In this talk, I will cover some of the recent progress. This will include improved electron and ion broadening, involving the creation of better collision models. There has also been recent advancement in the field of highly-magnetized plasmas. Plasma processes in magnetized plasmas are severely under-developed; this talk will present the first electron-atom collisions in strong magnetic fields.
Dr. Liam Stanton
San Jose State University
Transport processes ar...
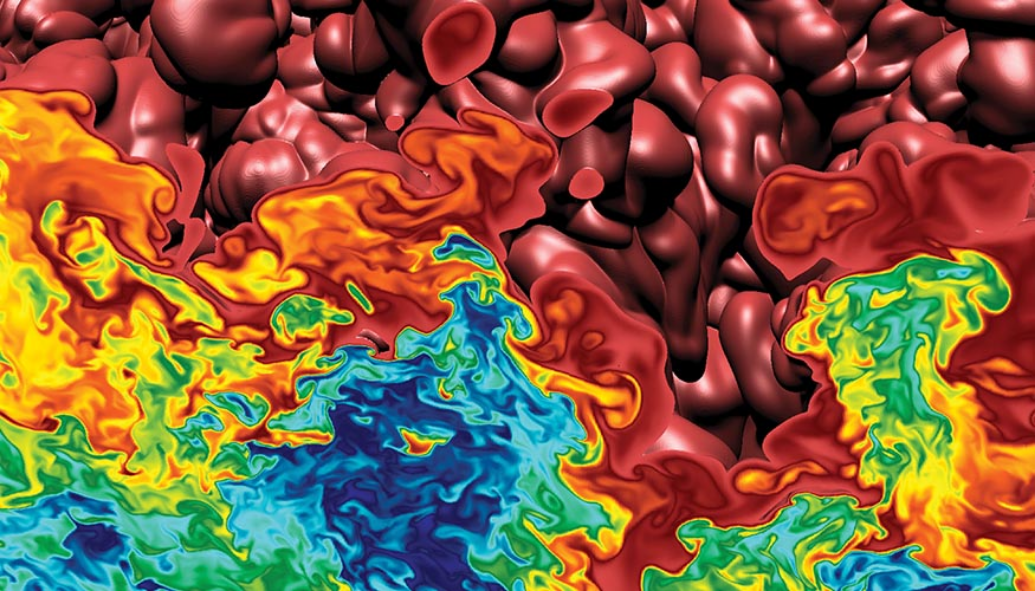
Dr. Liam Stanton
San Jose State University
Transport processes are important to accurately quantify the hydrodynamic description of plasmas, where these processes are typically captured through transport coefficients that relate the flux of one quantity in terms of the gradient in another. This talk will explore the extent to which simple screening models for particle interactions can capture the transport processes of non-ideal plasmas, such as the dense plasmas found in inertial confinement fusion (ICF) experiments. We have developed a simplified but effective potential approach that borrows from both the Boltzmann and Lenard-Balescu frameworks that yields accurate and computationally efficient fits for all of the relevant cross-sections and collision integrals needed to construct transport coefficients. Our results, which span the parameter regimes relevant to ICF, have been validated with molecular dynamics (MD) simulations for self-diffusion, inter-diffusion, and viscosity, with promising comparisons to experiments as well. MD simulations have also been used to examine the underlying assumptions of this effective Boltzmann approach through a categorization of behaviors of the velocity autocorrelation function. While this model was originally intended for ionic transport, the model can be applied to transport dominated by electronic interactions as well, such as thermal and electrical conductivity. Finally, the model can be extended to include dynamic screening effects for velocity-resolved transport processes like stopping power.
Dr. Mark B. Chadwick
Los Alamos National Laboratory
I describe the developing knowledge of fusion cross sections from 1934 through t...
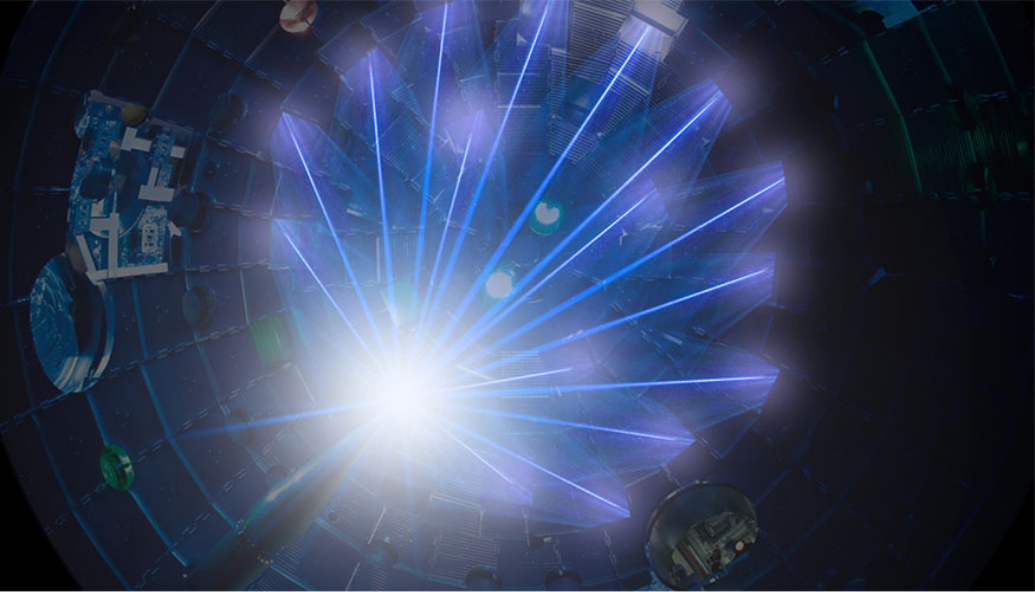
Dr. Mark B. Chadwick
Los Alamos National Laboratory
I describe the developing knowledge of fusion cross sections from 1934 through the first thermonuclear tests fielded in the Pacific from 1951–1952. At Oppenheimer's July 1942 Berkeley “luminaries” conference, Konopinski had suggested that the cross section for DT could be large, and although Teller described this as an inspired guess, I provide evidence instead suggesting that Konopinski knew of a 1938 measurement (by A. Ruhlig) that secondary DT reactions were “exceedingly probable.” Bethe's direction that the DT cross section should be measured at Purdue University in 1943 led to the remarkable and unexpected finding that the DT cross section exceeds DD by a factor of one hundred. This was a game-changing result, making Teller's dream—the terrestrial production of fusion energy— feasible. A description is given of the Manhattan Project’s 1945–1946 DD and DT measurements. These Bretscher Los Alamos experiments were the first to extend to very low energies in the 6–50 keV range that are needed in applications and to identify, characterize, and document the 3/2+ “Bretscher state” responsible for the resonant enhanced DT cross section. I end with the high accuracy (<2%) “APSST” measurements, 1951–1952.
Dr. David Turnbull
Laboratory for Lasers Energetics
University of Rochester
It is well known that radiati...
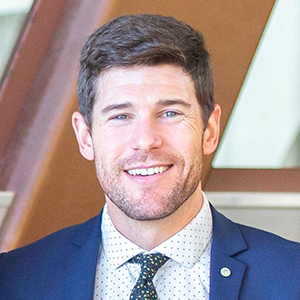
Dr. David Turnbull
Laboratory for Lasers Energetics
University of Rochester
It is well known that radiation-hydrodynamic simulations do not predict the results of integrated implosions with perfect fidelity. There are significant evidence points to deficiencies in the treatment of laser propagation through plasma. Here we review recent experimental results, studying key aspects of laser propagation: inverse bremsstrahlung absorption and susceptibility to beam spray. In both cases, the data indicate that certain commonly used models can fail to capture beam propagation by wide margins.
Dr. Maria Gatu-Johnson
Massachusetts Institute of Technology
Thermonuclear reaction rates and nuclear processes ...
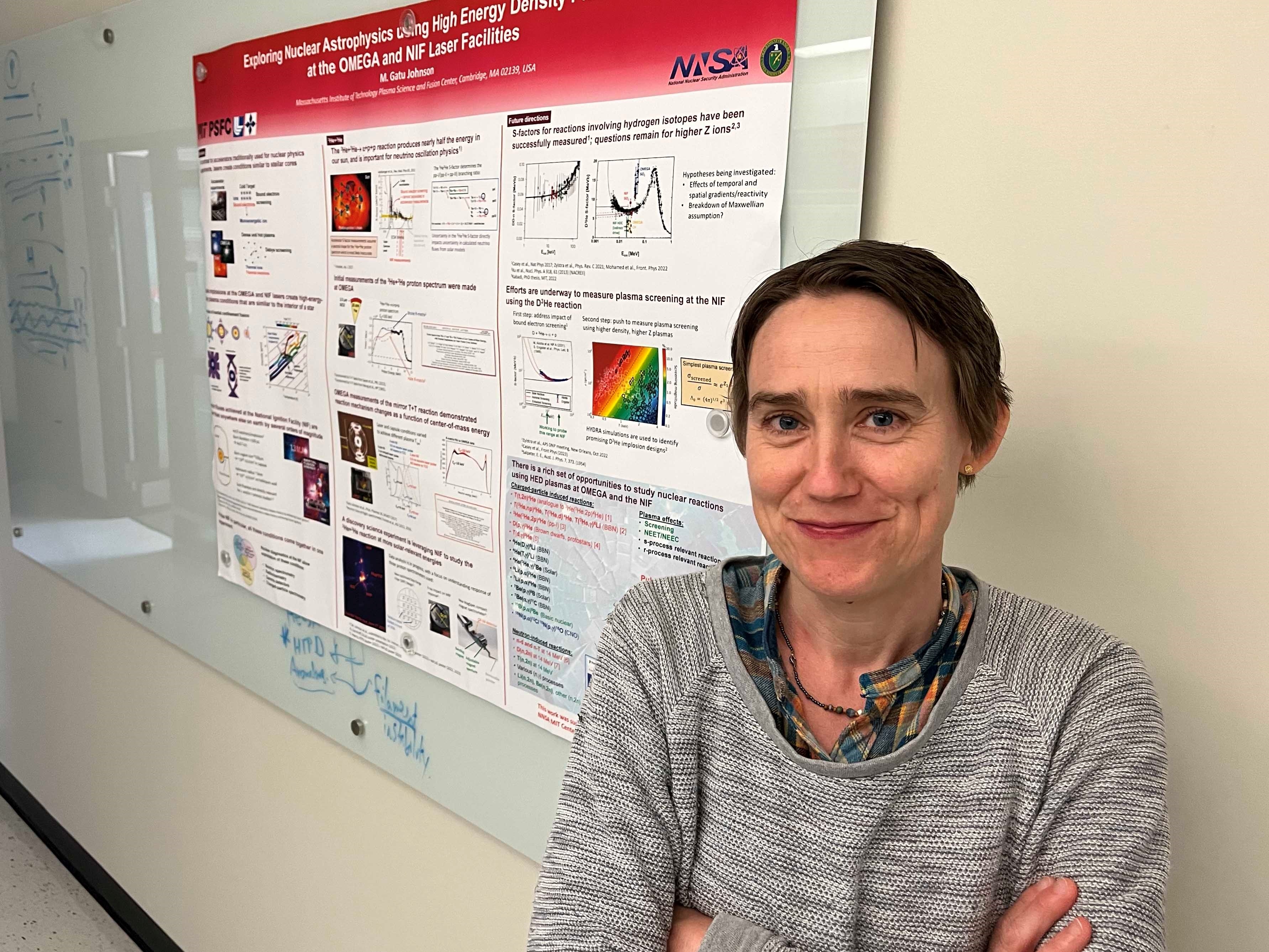
Dr. Maria Gatu-Johnson
Massachusetts Institute of Technology
Thermonuclear reaction rates and nuclear processes have traditionally been explored by means of accelerator experiments, which are difficult to execute at conditions relevant to stellar or big bang nucleosynthesis. High energy density (HED) plasmas generated using lasers, e.g., such as the inertial confinement fusion platform at the NIF and OMEGA laser facilities, more closely mimic astrophysical environments in several ways, including with thermal distributions of reacting ions as opposed to mono-energetic ions impinging on a cold target; stellar-relevant plasma temperatures and densities; and neutron flux densities not found anywhere else on Earth. This talk will present some initial nuclear astrophysics-relevant results for the T+T and solar 3He+3He reactions using this platform to illustrate the possibilities and describe ongoing efforts to study the 3He+3He reaction at stellar-relevant conditions at the NIF and with unprecedented data quality at OMEGA. Future directions, including platform developments to study CNO-cycle-relevant reactions including higher Z ions and to study neutron capture on s-process branching point nuclei will also be discussed. The work was supported in part by the US DOE, LLE, and LLNL.
Dr. Heather Whitley
Lawrence Livermore National Laboratory
Experiments in high energy density science (HEDS) have practical app...
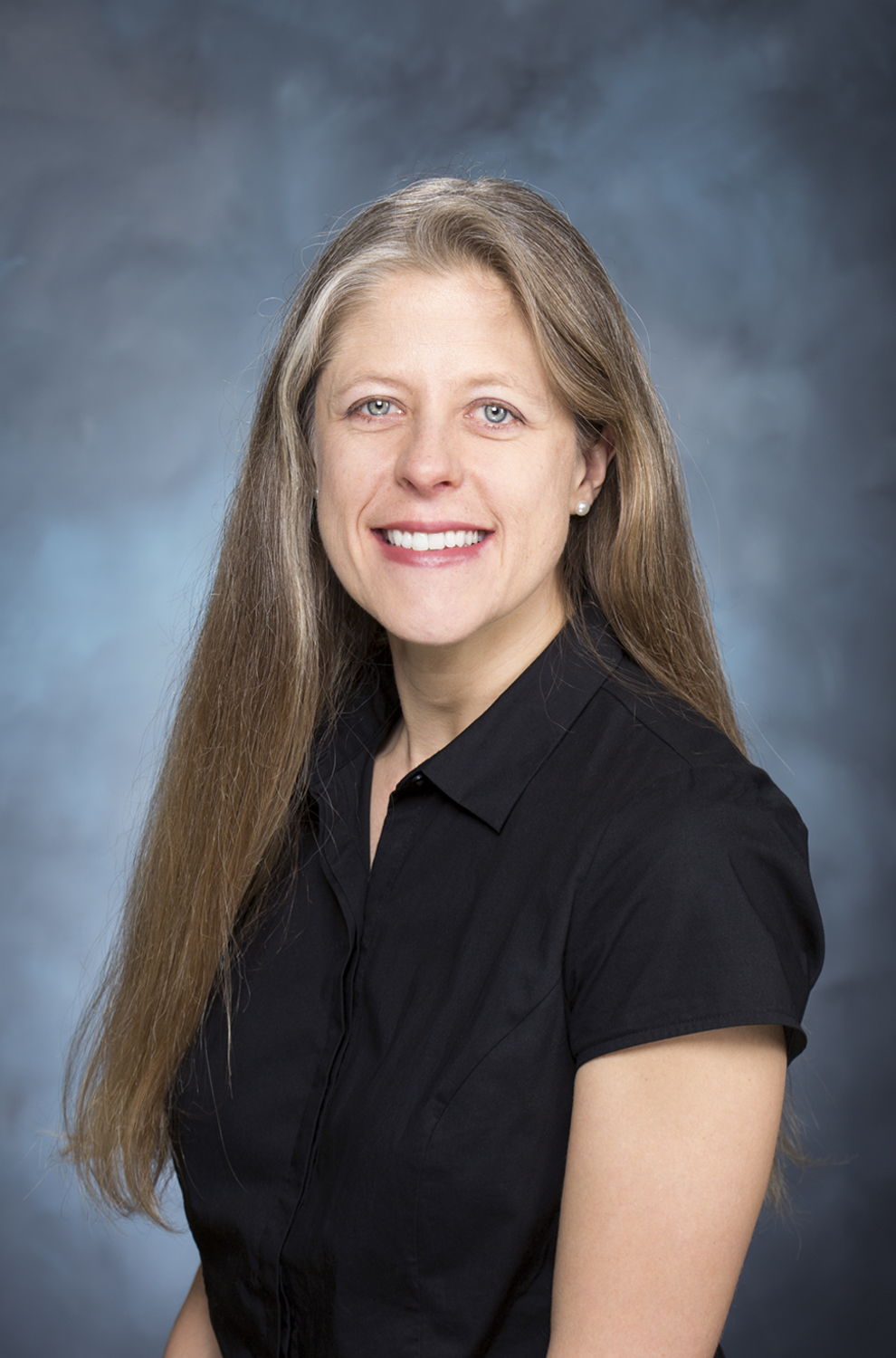
Dr. Heather Whitley
Lawrence Livermore National Laboratory
Experiments in high energy density science (HEDS) have practical applications for society ranging from studies that support the development of fusion energy platforms to providing scientific data that supports sustainment of the US nuclear deterrent. HEDS more broadly encompasses the study of matter at extreme temperatures and densities, ranging from those relevant to planetary science and stellar evolution to those created in inertial confinement fusion experiments. In this presentation, I will give an overview of ongoing experiments in materials, plasma, and nuclear science at the National Ignition Facility, including a few examples of how ignition opens new possibilities for expanding our basic science experiments.
Dr. Zachary Johnson
Michigan State University
Modeling the physics of high...

Dr. Zachary Johnson
Michigan State University
Modeling the physics of high-energy density plasmas (HEDP) is of critical importance to fusion technology and in diverse astrophysical settings. In particular, microscopic closure information in the form of equations of state and transport coefficients are needed to have robust predictive capability. In general, these processes tend to be too integrated in typical HEDP experiments (NIF, Omega, Z) to isolate for validation purposes. Much of this physics, however, depends on dimensionless parameters that can also be achieved in much smaller scale experiments. In particular, the laser-breakdown of noble gases achieves strong coupling in a regime with largely classical electrons and moderate pressures. In this talk, we focus on laser breakdown experiments done at UCLA and describe our efforts to model this system with two temperature hydrodynamics. We explored the sensitivity of this experiment to models for electron-ion thermalization rates, the equation of state, and energy transport. We conclude with an outlook for the promising future directions for laser breakdown plasmas.
Dr. Atilla Cangi
Center for Advanced Systems Understanding
Helmholtz-Zentrum Dresden-Rossendorf (HZDR), Germany
In this talk, I will present two ...
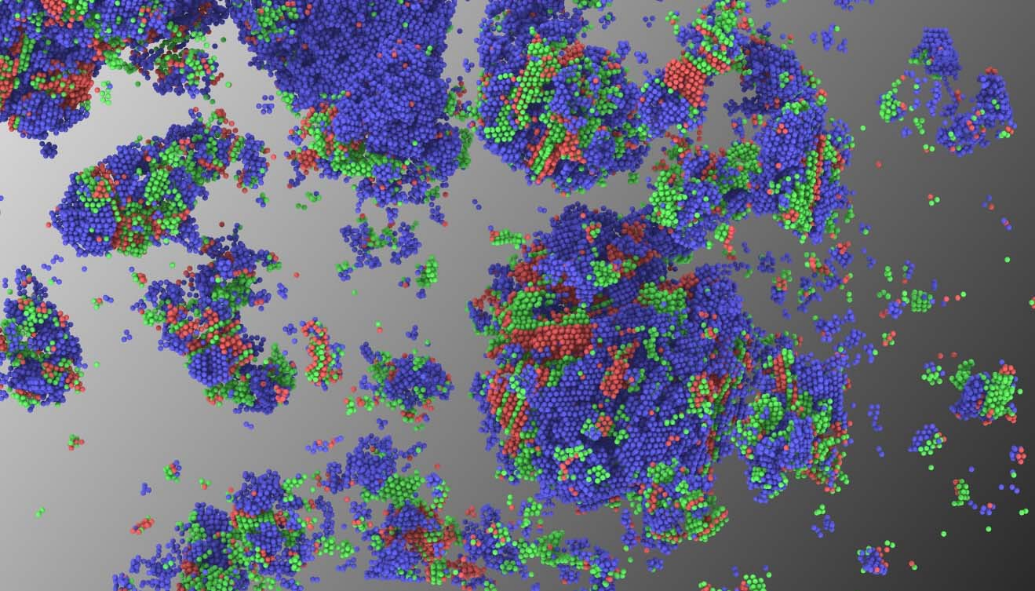
Dr. Atilla Cangi
Center for Advanced Systems Understanding
Helmholtz-Zentrum Dresden-Rossendorf (HZDR), Germany
In this talk, I will present two lines of our research. In the first part, I will discuss the application of time-dependent density functional theory (TDDFT) in modeling observables induced in matter under extreme conditions, such as the electron loss function relevant to scattering experiments with X-ray free electron lasers and the electrical conductivity in metals. In the second part, I will highlight our recent progress in leveraging Artificial Intelligence (AI) to enhance the efficiency of electronic structure calculations. Specifically, I will focus on our efforts to accelerate Kohn-Sham density functional theory calculations at finite temperatures by integrating deep neural networks into the Materials Learning Algorithms framework. Our results demonstrate significant improvements in calculation speed for metals up to their melting point. Additionally, our implementation of automated machine learning has led to substantial savings in computational resources for identifying optimal neural network architectures, paving the way for large-scale AI-driven investigations. Lastly, I will present our latest breakthrough, showcasing the capability of neural-network-driven electronic structure calculations for systems containing over 100,000 atoms.