The Center invites HED science researchers to deliver presentations as part of our weekly seminar series for LLNL staff, postdocs, and interns.
All presentations are the work of the speakers and owned by their respective institutions. We thank the speakers for permission to post their work here. To watch featured seminars, please visit the Livermore Lab Events YouTube channel.
If you are interested in delivering a seminar, we invite you to contact Federica Coppari, seminar series chair, at coppari1 [at] llnl.gov (coppari1[at]llnl[dot]gov)
Mike Campbell
Laboratory for Laser Energetics, University of Rochester
The Richtmyer-Meshkov insta...
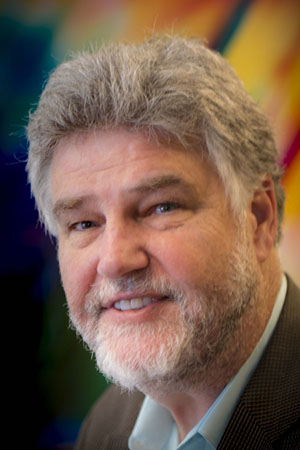
Mike Campbell
Laboratory for Laser Energetics, University of Rochester
The Richtmyer-Meshkov instability (RMI) develops upon impulsive acceleration of the interface between fluids of different acoustic impedance. The RMI consists in the unbounded growth of any perturbations initially present on the interface, and it is a consequence of the baroclinical deposition of vorticity on the interface. Eventually, the interface develops into a turbulent layer leading to the mixing of the two fluids. Flows of this type occur in nature and in man-made settings, over very large ranges of length, time, and energy scales: from experiments for the attainment of inertial confinement fusion (ICF), to supersonic combustion systems, to supernovae explosions. Shock-induced mixing has very negative effects in the case of ICF while it holds great potential to improve the combustion processes in the case of hypersonic engines. Two of the fundamental parameters that govern these phenomena are the Atwood number (measuring the original density contrast) and the Mach number. The experiments described here take place in a vertical shock tube with large, square internal cross section. The initial condition consists of a shear layer between helium and argon. A downward-propagating planar shock wave of Mach number 1.6 or 2.2 accelerates the interface. Measurements of 2-D concentration and velocity fields in a vertical cross section of the flow are obtained using planar laser-induced fluorescence (PLIF) and particle image velocimetry (PIV), respectively. Field statistics and integral quantities are extracted from these data and turbulence and mixing parameters are evaluated. From them, the outer scale Reynolds number is estimated to reach between 10,000 and 20,000 at the late times, indicating that the flow has crossed the threshold of fully developed turbulence.
Dawn Shaughnessy
Lawrence Livermore National Laboratory
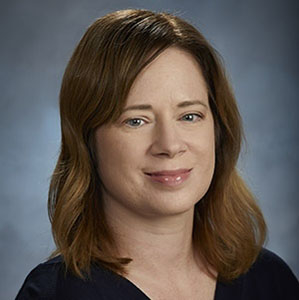
Dawn Shaughnessy
Lawrence Livermore National Laboratory
The National Ignition Facility (NIF) is the world’s largest laser and is a major contributor to the Stockpile Stewardship Program conducting state of the art science in high energy density physics including fusion research. Developing the mission, science, technology, and support for projects of the scale of NIF is a demanding and multifaceted enterprise. There are many lessons to be learned from the NIF experience that can be applied in the quest to secure any future large-scale facility. This presentation will include a historical perspective on inertial confinement fusion (ICF) at Lawrence Livermore National Laboratory and the Stockpile Stewardship Program that motivated the NIF and the scientific and political strategy that ultimately secured the Facility.
Maria Gomez
Mount Holyoke College
Finding long range conduc...
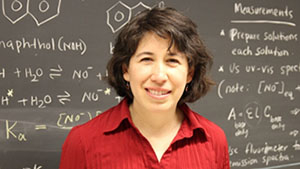
Maria Gomez
Mount Holyoke College
Finding long range conduction pathways is challenging when the system includes both fast frequency modes needing short time step force integration and slow frequency modes requiring long time samples. Methods such as kinetic Monte Carlo (kMC) avoid the integration of steps and instead use probabilities to choose the next moves and advanced the clock based on the move chosen. Nevertheless, extracting fast conduction mechanisms between traps can be challenging. Centrality methods based on the number of steps to return to key sites or vertices in a graph have been used to identify the most central areas in a graph. However, physical systems with traps and highways have steps with distinctly different barriers making steps non-equivalent. This seminar reviews both traditional and new graph theory schemes for finding long range pathways with a special focus on a centrality measure that instead of using the number of steps to return to sites considers the time of first returns and its applications to proton conduction in doped perovskites. In these systems shown below a single image including all proton binding sites with the most central sites shown in darker shades of grey shows both trapped regions and fast conduction highways.
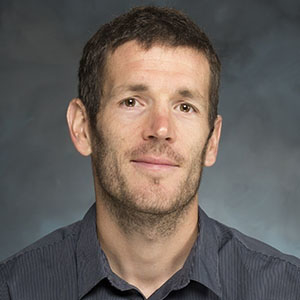
Marius Millot
Lawrence Livermore National Laboratory
We will stream the documentary movie Jupiter Revealed from the BBC's Horizon show. This film describes new discoveries about the planet Jupiter following the success of the NASA Juno probe mission and how these discoveries help us better understand how planet form and evolve. One of the segments was filmed at the National Ignition Facility in Livermore. This segment highlights a series of Discovery Science experiments conducted at NIF to explore the exotic properties of metallic Hydrogen.
Felicie Albert
Lawrence Livermore National Laboratory
Parti...
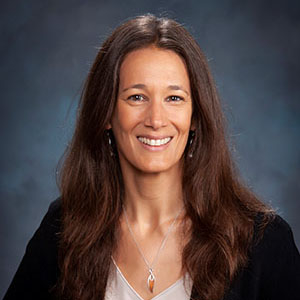
Felicie Albert
Lawrence Livermore National Laboratory
Particle accelerators have been revolutionizing discoveries in science, medicine, industry, and national security for more than a century. An estimated 30,000 particle accelerators are active around the world. In these machines, electromagnetic fields accelerate charged particles, such as electrons, protons, ions, or positrons to velocities nearing the speed of light. Although their scientific appeal will remain evident for many decades, one limitation of the current generation of particle accelerators is their tremendous size (typically a mile long) and cost, which often limits access to the broader scientific community. A plasma is a neutral medium composed of negatively charged free electrons and positively charged ions. Plasma can sustain electrical fields three orders of magnitude higher than that of conventional particle accelerators. Acceleration of electrons in laser-driven plasmas has been drawing considerable attention over the past decade. These laser wakefield accelerators promise to dramatically reduce the size of accelerators and revolutionize applications in medicine, industry, and basic sciences.
Tammy Ma
Lawrence Livermore National Laboratory
The National Ignit...
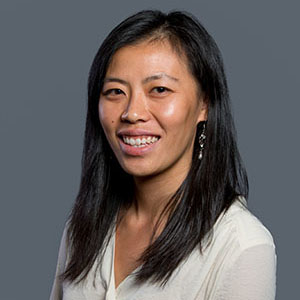
Tammy Ma
Lawrence Livermore National Laboratory
The National Ignition Facility (NIF) at the Lawrence Livermore National Laboratory (LLNL) is the world’s largest and most energetic laser system. The NIF is built to create very extreme states of matter—similar to those found in the interiors of stars and planets. Here, scientists and engineers are working hard to demonstrate sustainable fusion burn—the same reaction that occurs in the sun—to one day harness as a source of limitless, clean energy.
Jeffrey Haack
Los Alamos National Laboratory
Designing effective m...
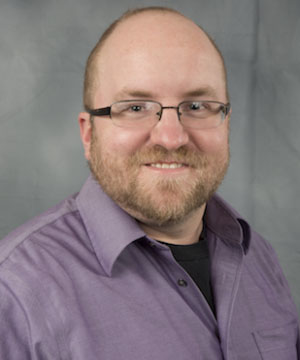
Jeffrey Haack
Los Alamos National Laboratory
Designing effective methods for multiscale simulation is a longstanding challenge. Our goal is to advance the state of the art for Machine Learning (ML) beyond sequential training and inference and facilitate scale bridging through novel techniques. Active Learning (AL) is a special case of semi-supervised ML in which a learning algorithm is able to interactively use the fine-scale model to obtain the desired outputs at new data points, making it ideal for concurrent scale-bridging. Our AL procedure will dynamically assess uncertainties of the ML model, query new fine scale simulations as necessary, and use the new data to incrementally improve our ML models. This capability will be demonstrated on two applications: transport in nanoporous media (e.g., for hydraulic fracturing) and inertial confinement fusion (ICF), validating against experimental data. Although the physics is quite dissimilar, both applications represent problems that suffer from inaccurate macro-scale predictions due to subscale physics that are ignored.
Andrea Kritcher
Lawrence Livermore National Laboratory
White dwarf...
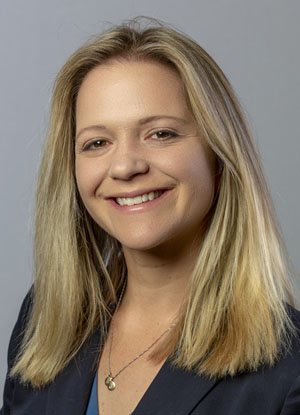
Andrea Kritcher
Lawrence Livermore National Laboratory
White dwarfs (WD) represent the final state of evolution for the vast majority of stars. Certain classes of white dwarfs pulsate, leading to observable brightness variations whose analysis with theoretical stellar models uniquely probes their internal structure. Modeling of these pulsating WD stars provides stringent tests of white dwarf models and a detailed picture of the outcome of the late stages of stellar evolution. However, these high energy density states are extremely difficult to access and diagnose in the laboratory and as a result theory is largely untested at these conditions. Here, we present equation of state (EOS) measurements of matter at pressures ranging from 100-450 million atmospheres, where the understanding of WD stars is sensitive to the EOS and where models show significant differences. We measure the pressure-density relationship along the principal shock Hugoniot of hydrocarbon to within five percent. The observed maximum compressibility is consistent with theoretical models that include detailed electronic structure and are used in calculations of WD stars and inertial confinement fusion (ICF) experiments to predict an increase in compressibility due to ionization of the inner core orbitals of carbon. We also find that detailed treatment of the electronic structure and the electron degeneracy pressure are required to capture the measured shape of the pressure-density evolution for hydrocarbon before peak compression.
Antonino Di Piazza
Max Planck Institute for Nuclear Physics
Quantu...
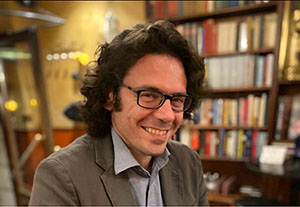
Antonino Di Piazza
Max Planck Institute for Nuclear Physics
Quantum electrodynamics (QED) is a well-established physical theory, and its predictions have been confirmed experimentally in various regimes and with extremely high accuracy. However, there are still areas of QED that deserve theoretical and experimental investigation—especially when physical processes occur in the presence of intense background electromagnetic fields, i.e., of the order of the so-called “critical” field of QED. In the presence of electromagnetic fields of such high strength, for example, even the vacuum becomes unstable and electron-positron pair production spontaneously occurs.
After a broad introduction on strong-field QED, the seminar will describe different regimes of this theory and introduce present and upcoming experimental efforts to test it under such extreme conditions. As a prominent theoretical example of open problems, the talk will focus on how the properties of the vacuum are altered by intense background electromagnetic fields. Unlike classical electrodynamics, QED predicts that electromagnetic fields also interact in vacuum through virtual electron-positron pairs. This is the physical origin of a number of so-called vacuum-polarization effects, which will be reviewed. Finally, the talk will discuss recent experimental suggestions to observe these effects by means of intense laser beams.
Zhandos Moldabekov
Al Farabi Kazakh National University
Methods fo...
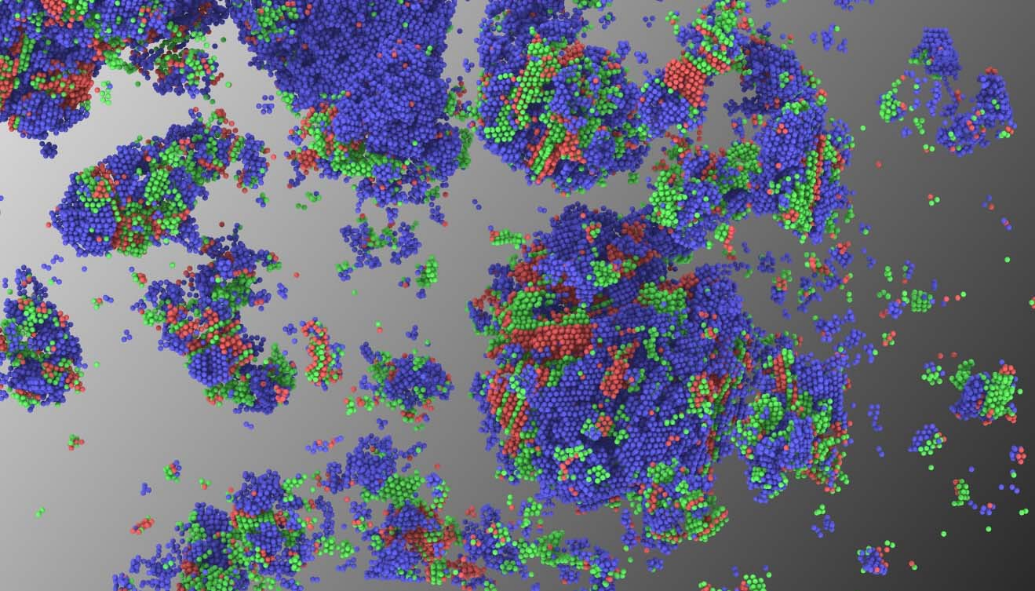
Zhandos Moldabekov
Al Farabi Kazakh National University
Methods for large-scale simulation of time-dependent features of quantum plasmas are crucial for fundamental and applied problems. The microscopic theory of quantum hydrodynamics (MQHD), which goes beyond the random phase approximation by employing ab initio data for dynamic local field corrections, will be presented. Quantum hydrodynamic theory is presented as the orbital averaging of the MQHD equations. The range of applicability of QHD is analyzed. Going beyond the gradient expansion approximation, a fully non-local Bohm potential is derived.
The results for the dynamic structure factor of ions at dense plasma and warm dense matter (WDM) conditions will also be presented. The applicability of the classical generalized hydrodynamics and of the QHD for the description of collective oscillations of ions will be discussed. Additionally, the results for the energy loss characteristics of electrons at WDM and dense plasma conditions, where the static local field correction from ab initio QMC simulations was used, will be presented. Finally, the first results for the friction coefficient acting upon ions due to electrons will be reported, where friction represents a correction to the Born-Oppenheimer approximation within Langevin dynamics simulation of ions.
Liam Stanton
San Jose State University
Computational models are fo...
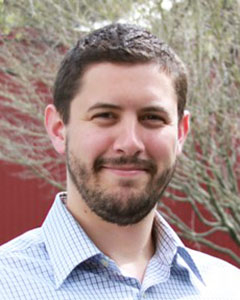
Liam Stanton
San Jose State University
Computational models are formulated in hierarchies of variable fidelity, often with no quantitative rule for defining the fidelity boundaries. This talk will explore the extent to which simple screening models for ionic interactions can capture both the equilibrium structure and transport processes of non-ideal plasmas, such as dense plasmas found in inertial confinement fusion (ICF) experiments and ultra-cold neutral plasmas (UNPs). We have developed a simplified effective potential approach within a Boltzmann-type framework that yields accurate and computationally efficient fits for all of the relevant cross-sections and collision integrals needed to construct transport coefficients. Our results, which span the UNP-to-ICF regimes, have been validated with molecular dynamics (MD) simulations for self-diffusion, inter-diffusion, viscosity, thermal conductivity, and stopping power with promising comparisons to UNP experiments as well. MD simulations have also been used to examine the underlying assumptions of this effective Boltzmann approach through a categorization of behaviors of the velocity autocorrelation function. In addition, we have constructed a dataset from a wide range of atomistic computational models to reveal the accuracy boundary between these higher-fidelity models and simpler screening models based on finite-temperature orbital-free density functional theory. The symbolic decision boundary is discovered by optimizing a support vector machine on the data through iterative feature engineering. This machine-learning approach reveals a symbolic rule that is independent of the computational method and directly reveals the central role of atomic physics in determining accuracy.
Robert Tipton
Lawrence Livermore National Laboratory
In 1955 John ...
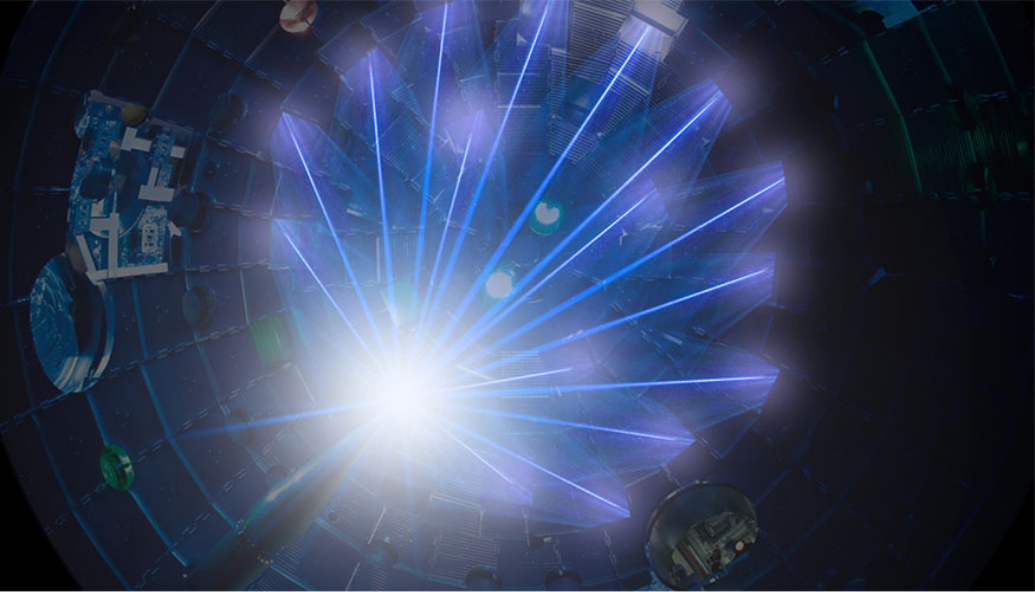
Robert Tipton
Lawrence Livermore National Laboratory
In 1955 John Lawson proposed a criterion of ignition in the context of magnetic fusion. Over the decades, Lawson’s original criterion has been generalized in several different ways for the case of Inertial Confinement Fusion (ICF). This talk will describe the different generalizations and demonstrate their effectiveness with numerical simulations. The simulations indicate our best shots on NIF have a generalized Lawson criterion which is about 80% of the expected ignition value.
Renata Wentzcovitch
Columbia University
Pressure and temperature i...
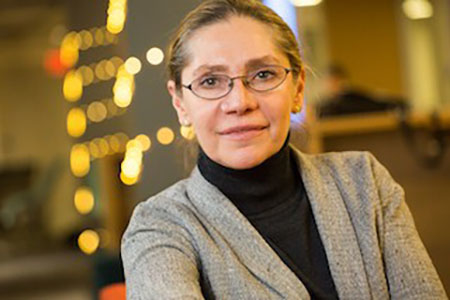
Renata Wentzcovitch
Columbia University
Pressure and temperature induced spin-state change in iron in lower mantle minerals is an unusual phenomenon with previously unknown consequences. High pressure and high temperature experiments have offered a wealth of new information about this class of materials problems, which includes the insulator to metal transition in Mott systems. I will discuss key experimental data, contrast them with our ab initio results and thermodynamic models, show the implications for fundamental phenomena taking place at the atomic scale and their macroscopic manifestations, and discuss potential geophysical consequences of this phenomenon.
David Strubbe
University of California, Merced
Many electronic-str...
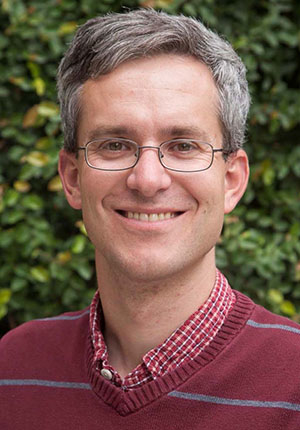
David Strubbe
University of California, Merced
Many electronic-structure studies of warm dense matter have focused on the end-point of compression, in total thermal equilibrium, or perhaps a partial equilibrium with different electronic and lattice temperatures. In compression experiments at laser facilities such as NIF, the shock speed can reach 10 km/s, which is equivalent to 10 Å/fs. This means that a direct calculation of the compression process itself is actually feasible with first-principles methods because the compression operates on the natural length and time scales of these methods, such as time-dependent density-functional theory (TDDFT). The seminar will discuss progress toward TDDFT simulations of ultrafast compression processes, assessing numerical and conceptual issues of electronic dynamics with a rapidly varying unit cell. It will also present results on changes in band structure and optical absorption spectra of diamond structure silicon under extreme pressure but low temperature, in which transitions take place from semiconductor to semimetal to metal on short timescales before the structure can change to the other phases of silicon favored at high pressure. Finally, the seminar will cover our work on non-additive kinetic energy functionals, which can provide a route to improved DFT-based average-atom or neutral pseudo-atom models for high energy density science. This work is being carried out as part of the Consortium for High Energy Density Science.
Daniel Casey
Lawrence Livermore National Laboratory
Laser-based in...
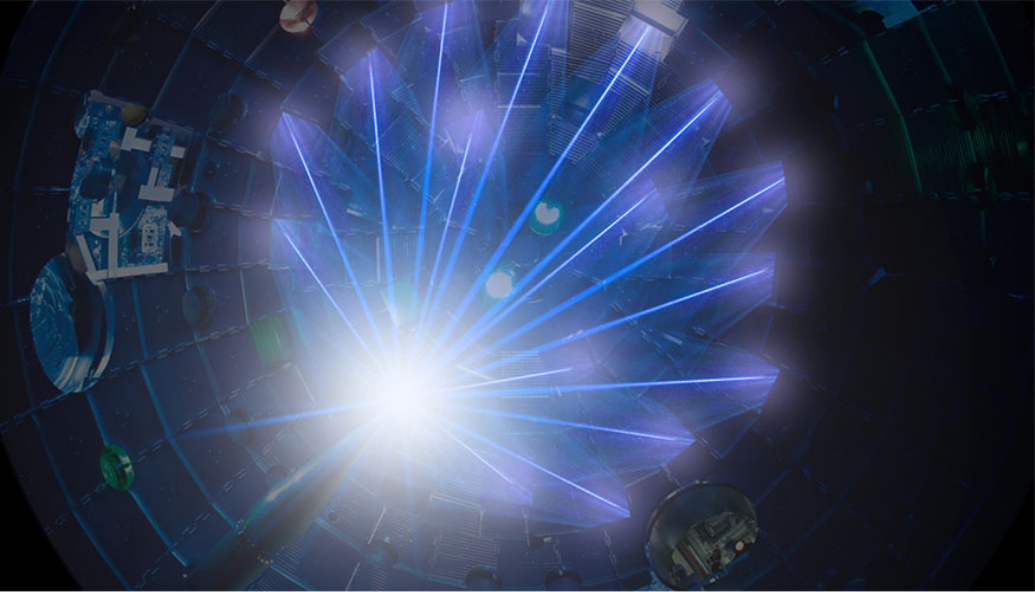
Daniel Casey
Lawrence Livermore National Laboratory
Laser-based inertial confinement fusion (ICF) implosions provide a path to study reactions in thermonuclear plasmas. However, ICF experiments have significant challenges not found in accelerator experiments. This talk explored how these issues can be overcome and ICF implosions can be used to make nuclear measurements in some specific circumstances. In particular, the method of yield ratios is used to infer astrophysical S-factors for two reactions using a third as a reference. The resulting data show excellent agreement with evaluations and prior accelerator data for these two reactions, bolstering confidence in this method. This technique is now being explored as a candidate for a future plasma-electron-screening experiment to attempt to observe enhancements to reaction rates in the presence of plasma electrons.
Baptiste Journaux
University of Washington
The presenter discussed...
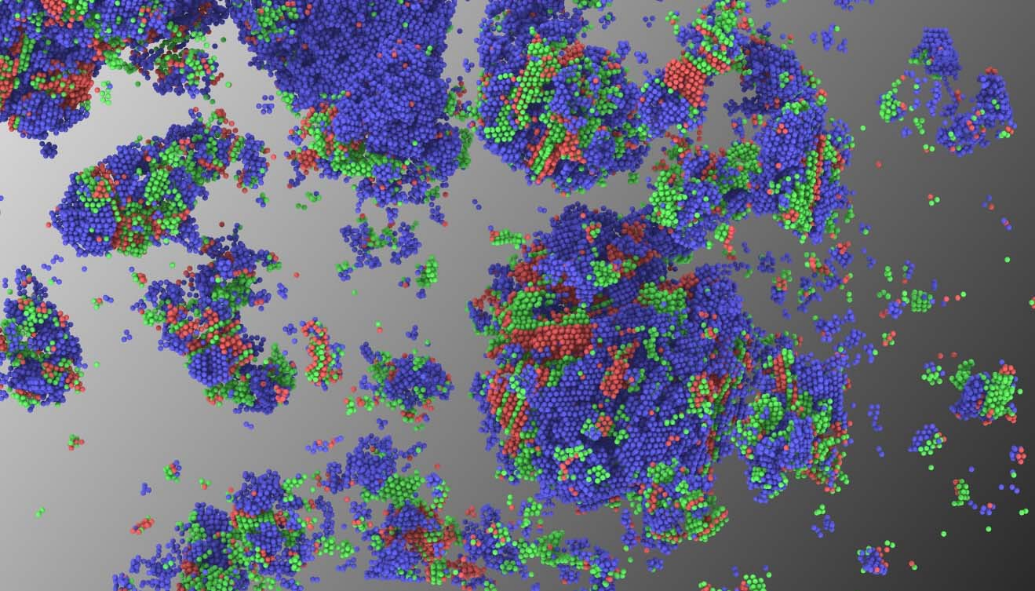
Baptiste Journaux
University of Washington
The presenter discussed his work related to studying the habitability of planetary interiors. The research team operated diamond anvil cell X-Ray diffraction experiments coupled with new advanced thermodynamic representation that allow scientists to accurately reproduce experimental data. This modular framework, based on local basis functions parameterization of a thermodynamic potential, allows accurate prediction of phase stability and realistic equilibrium thermodynamics in stable and metastable regime, and can be used in the representation of UHP experimental results.
Yitzhak Maron
Weizmann Institute of Science, Israel
The presenter ...
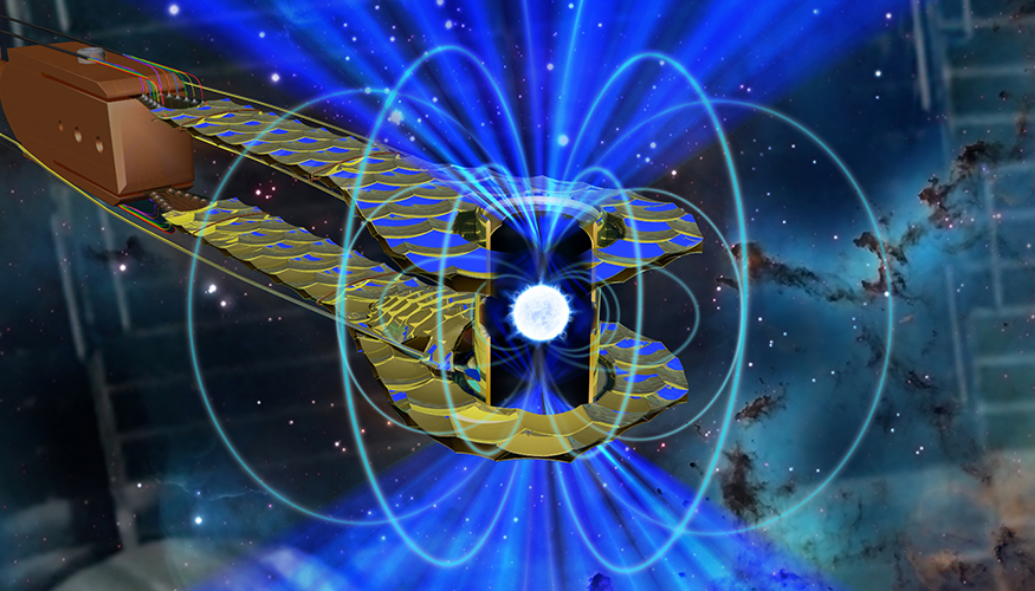
Yitzhak Maron
Weizmann Institute of Science, Israel
The presenter discussed an experimental method to determine the ion temperature based on the effect of ion coupling on the Stark line shapes. It was implemented on a Z-pinch stagnation, confirming that the ion thermal energy is small compared to the imploding-plasma kinetic energy, where most of the latter is converted to hydromotion. The seminar also included a discussion of the Zeeman Effect induced by intense laser light, with the presenter describing an analysis of spectral line shapes of hydrogen-like species subjected to fields of electromagnetic waves showing that the magnetic component of an electromagnetic wave may significantly influence the spectra. In particular, the Zeeman Effect induced by powerful visible or infrared lasers can be experimentally observed.
Damian Swift
Lawrence Livermore National Laboratory
In real-world ...
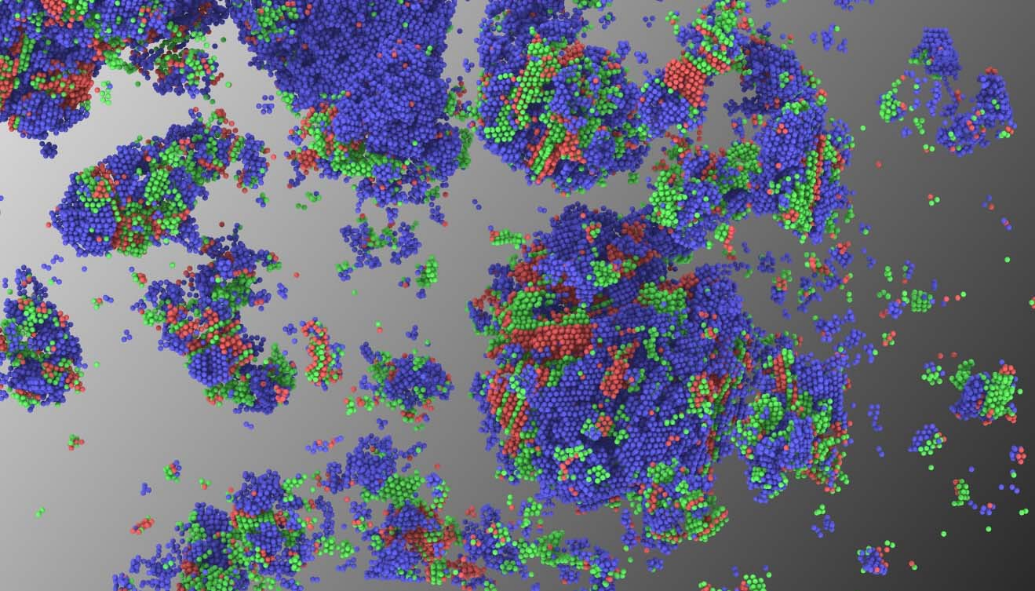
Damian Swift
Lawrence Livermore National Laboratory
In real-world situations such as in stars, the behavior of matter at extreme conditions can be very sensitive to variations between widely used equation of state (EOS) models. The presenter discussed work done by his team to develop experimental platforms accessing the EOS and atomic physics of matter in stellar regimes. Shock states up to ~80 TPa can be deduced from spherically converging shocks at LLNL’s National Ignition Facility, and the team recently improved the analysis to reduce uncertainties and infer off-Hugoniot data.
Bruce Cohen
Lawrence Livermore National Laboratory
The presenter d...
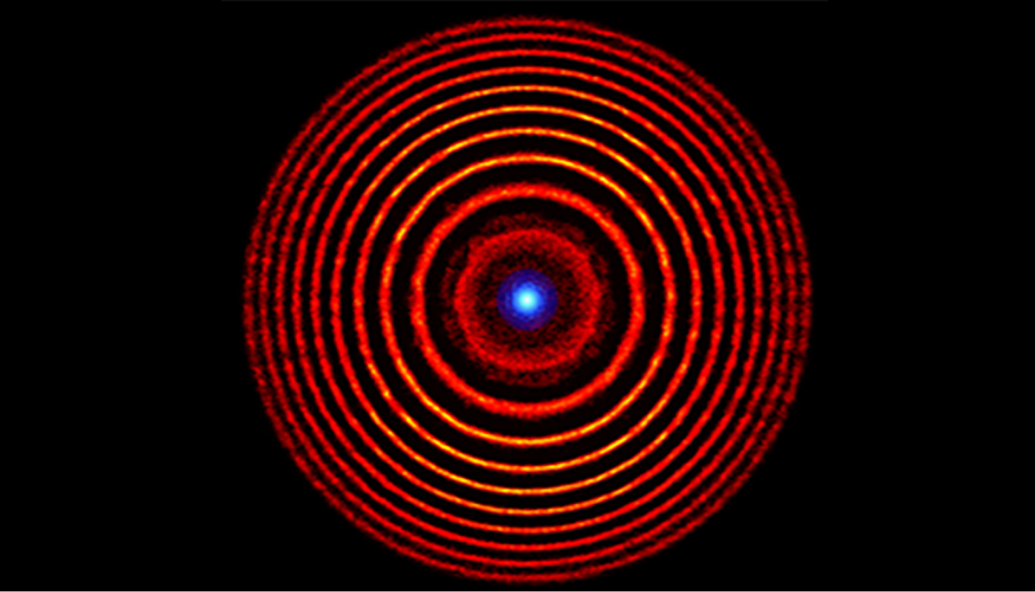
Bruce Cohen
Lawrence Livermore National Laboratory
The presenter discussed his perspective on the development and application of computational plasma physics to plasmas in nature and in the laboratory. The examples described illustrated both fundamental plasma phenomena and the behavior of laboratory plasmas. Some of the specific examples included simulations of micro-instabilities in magnetic mirror, tokamak, and spheromak plasmas; laser-plasma interactions; and Knudsen-layer phenomena as it affects fusion performance. The examples also illustrated the development of models and algorithms that are well suited to simulating the phenomena of interest.
Fridolin Weber
San Diego State University and University of California, San Diego
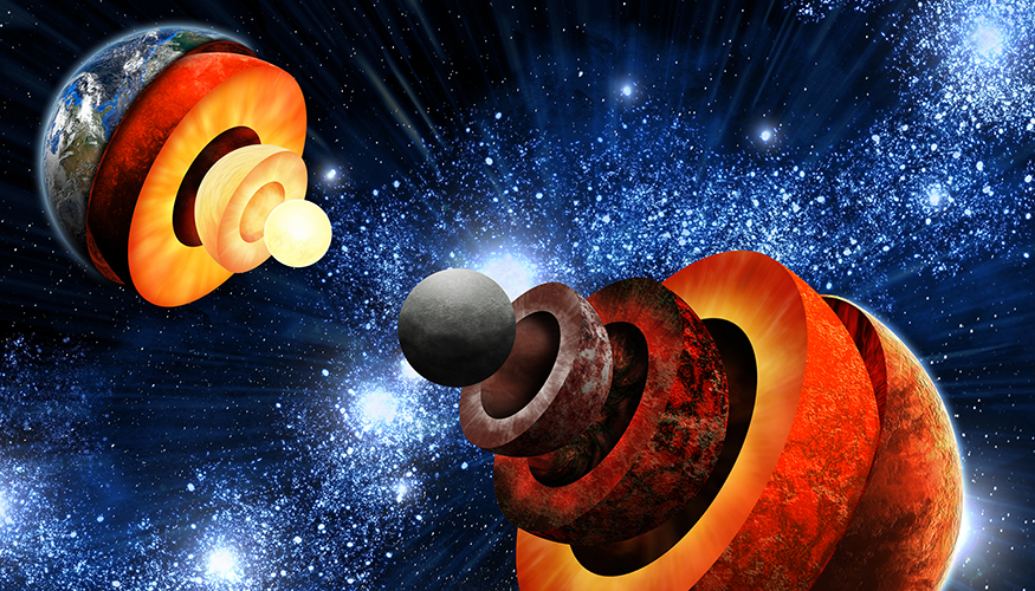
Fridolin Weber
San Diego State University and University of California, San Diego
In this talk, the presenter provided an overview of our current understanding of the composition of neutron stars. Models for the equation of state of dense neutron star matter were also presented, which are constrained by the latest nuclear and astrophysical data. Particular emphasis was put on quark de-confinement in the core regions of neutron stars. Finally, the phase diagram of hot and dense proto-neutron star matter was discussed, and possible instabilities in such matter were pointed out.
Frank Graziani
Lawrence Livermore National Laboratory
In this talk...
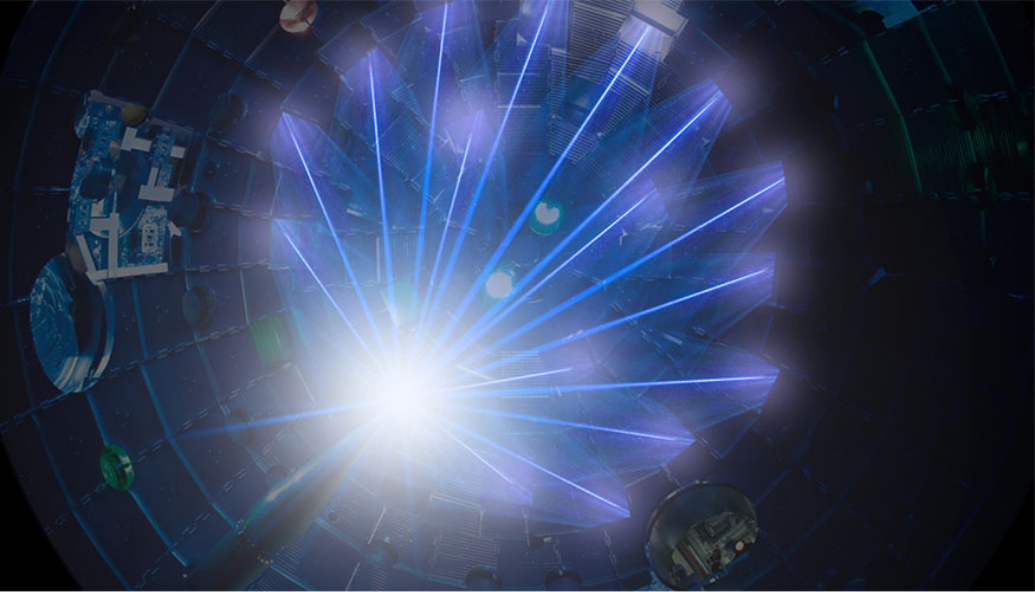
Frank Graziani
Lawrence Livermore National Laboratory
In this talk, the director of LLNL’s HED Science Center presented the 2019 year in review and looked at what lies ahead in 2020. In 2019, the Center saw growth in its education programs, selected a deputy director, established a new HEDS Center postdoctoral fellowship, participated in LLNL’s Ambassador program, established closer ties with Japan’s HED efforts, delivered a vibrant seminar series, hosted summer student and thesis programs, and engaged in collaborations with many academic partners, including several minority serving institutions. The presenter also discussed plans for 2020, including a consortium of universities engaged in HED science education, selection of the HEDS Center postdoctoral fellow, hosting sabbatical programs, and various outreach programs.
Sofia Quaglioni
Lawrence Livermore National Laboratory
An overarch...
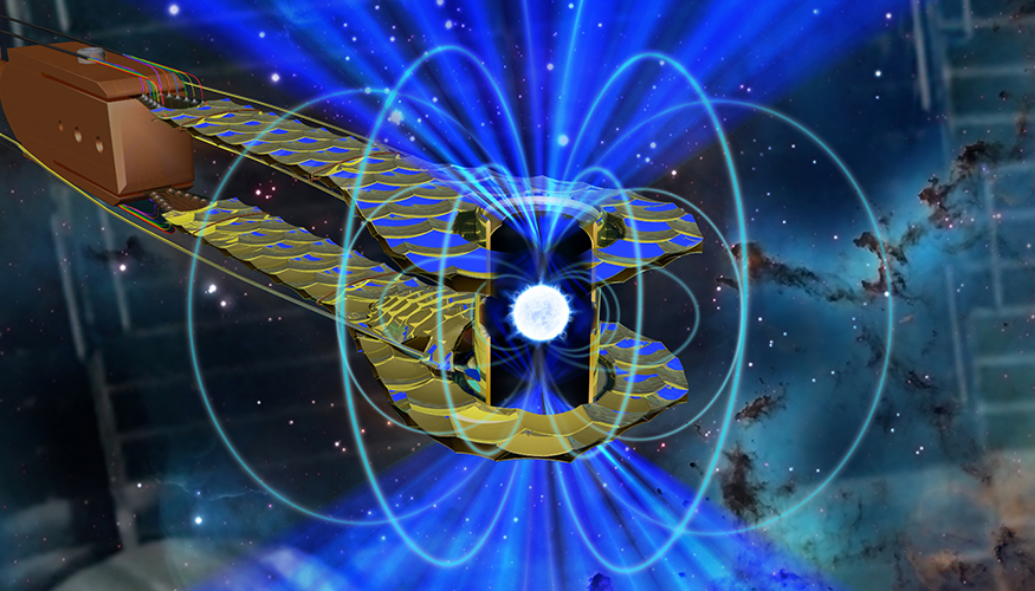
Sofia Quaglioni
Lawrence Livermore National Laboratory
An overarching goal of nuclear physics is to arrive at the comprehensive understanding—in terms of the laws of quantum mechanics and the underlying theory of the strong force (quantum chromodynamics)—of atomic nuclei and their interactions, and to use this understanding to accurately predict nuclear properties that play a fundamental role in explaining the inner workings of the Universe or are critical to national security. The presenter discussed first-principles calculations of nuclear structural and reaction properties to predict thermonuclear reaction rates of interest for fusion energy technology and stellar nucleosynthesis.
Hui Chen
Lawrence Livermore National Laboratory
Intense lasers are...
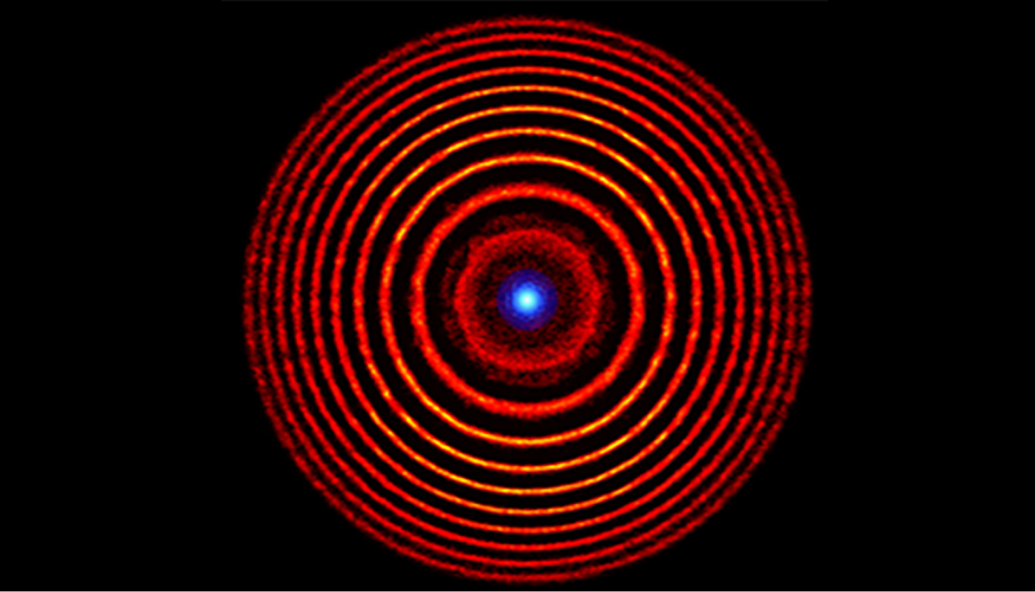
Hui Chen
Lawrence Livermore National Laboratory
Intense lasers are now the brightest source of relativistic electron-positron pair jets in the laboratory—up to 1012 of highly relativistic pairs are routinely produced in experiments at LLNL and other high power, short pulse lasers with energies from 100-2000 J. This talk reviewed experimental results to date. In addition, the presenter discussed the near-future in this research area, exploiting laser-produced pair jets for fundamental plasma physics studies, for diagnosing high-energy-density physics, and for laboratory experiments mimicking the physics processes in various astrophysical observations including Gamma Ray Bursts.
Federica Coppari
Lawrence Livermore National Laboratory
Since Brid...
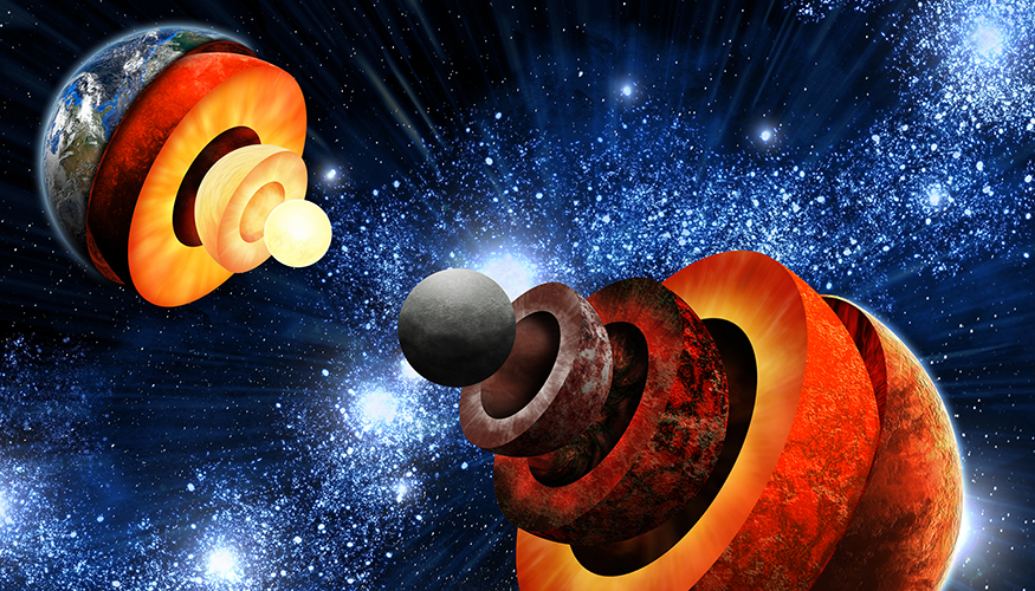
Federica Coppari
Lawrence Livermore National Laboratory
Since Bridgman’s discovery of five ice phases in 1912, studies on the extraordinary polymorphism of H2O have documented 17 crystalline and several amorphous structures, as well as metastability and kinetic effects. Particularly intriguing is the prediction that water becomes superionic—with liquid-like hydrogens diffusing through the solid lattice of oxygen—when subjected to extreme pressures exceeding 100 GPa and temperatures above 2,000 K. Optical measurements along the Hugoniot curve of ice VII showed evidence of superionic conduction but did not confirm the microscopic structure of superionic ice. The presenter discussed recent experiments at the OMEGA Laser that used shockwaves to simultaneously compress and heat liquid water samples to 100–400 GPa and 2,000–3,000 K. In-situ x-ray diffraction shows that under these conditions, water solidifies within a few nanoseconds into nanometer-sized ice grains that exhibit unambiguous evidence for the crystalline oxygen lattice of superionic water ice. These data allow us to document a temperature- and pressure-induced phase transformation from a body-centered-cubic ice phase to a novel face-centered-cubic, superionic ice phase.
Thomas Gomez
Sandia National Laboratories
Broadening of atomic lin...
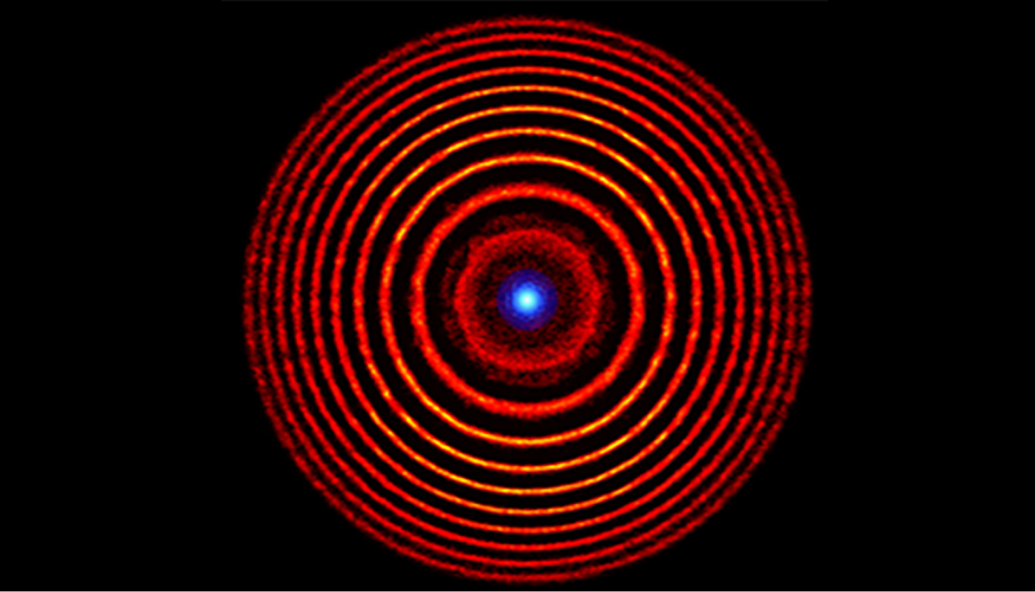
Thomas Gomez
Sandia National Laboratories
Broadening of atomic lines is caused by a time-dependent perturbation on the atom due to the plasma ions and electrons surrounding it. The relative importance of each broadening mechanism depends on transitions and conditions. The broadening due to slow-moving ions is well understood compared with the broadening from electrons due to more complex time-dependent quantum-mechanical interaction with the radiating atom. In fact, there is a long-standing discrepancy between modeled and measured line width for these Li-like ions; this is known as the isolated-line problem. The presenter discussed common approximations and limitations in electron-broadening calculations, as well as the impact of our recent investigations on the isolated-line problem.
Yuri Litvinov
GSI Helmholtzzentrum für Schwerionenforschung, Germany
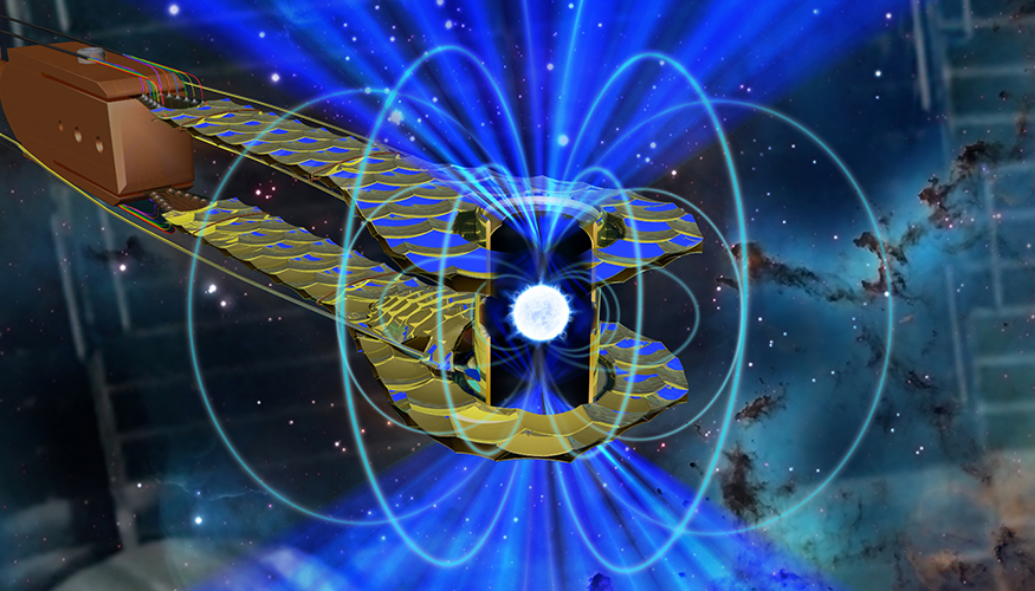
Yuri Litvinov
GSI Helmholtzzentrum für Schwerionenforschung, Germany
Storage of freshly produced radioactive particles in a storage ring enables efficient use of the same rare ion multiple times. Employing heavy-ion storage rings for precision physics experiments with highly-charged ions (HCI) is a rapidly developing field of research. At the heavy-ion storage ring ESR at GSI, construction of dedicated heavy-ion storage rings have enhanced experimental capabilities by enabling stored and cooled secondary HCIs in the previously inaccessible low-energy range. The presenter highlighted research programs at heavy-ion storage rings, including physics experiments at the intersection of atomic, nuclear, and possibly plasma physics.
Gilles Kluth
French Alternative Energies and Atomic Energy Commission, France
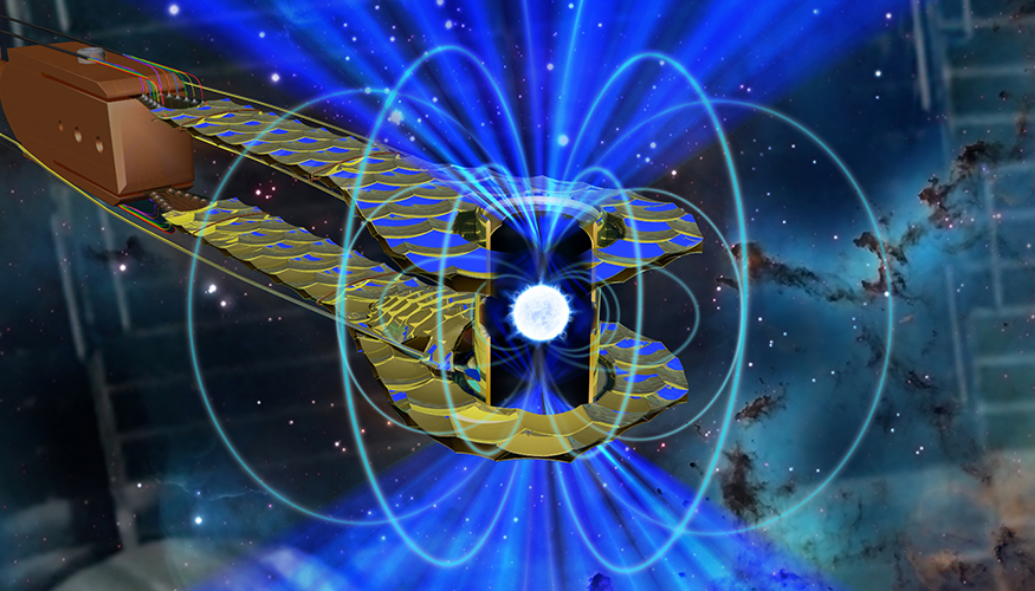
Gilles Kluth
French Alternative Energies and Atomic Energy Commission, France
The presenter discussed the team’s work developing new techniques to accelerate radiation hydrodynamics simulations. The ultimate goal is to develop a fast representation of detailed atomic physics packages to give absorption coefficients, emissivities, and ionization states. The fast representation—in this case a deep neural network—can be called in place of a traditional physics package. The neural network is dramatically faster and uses substantially less memory. The team examined the NLTE physics of mid-Z materials for ICF simulations as a test application. They used Cretin for in-line collisional-radiative computations in the rad-hydrocode HYDRA, and they trained a deep neural network on a set of Cretin data under a broad set of plasma conditions. Finally, they replaced the in-line atomic physics computation in HYDRA with the well-trained neural network accelerator.
Thomas Schenkel
Lawrence Berkeley National Laboratory
The presente...
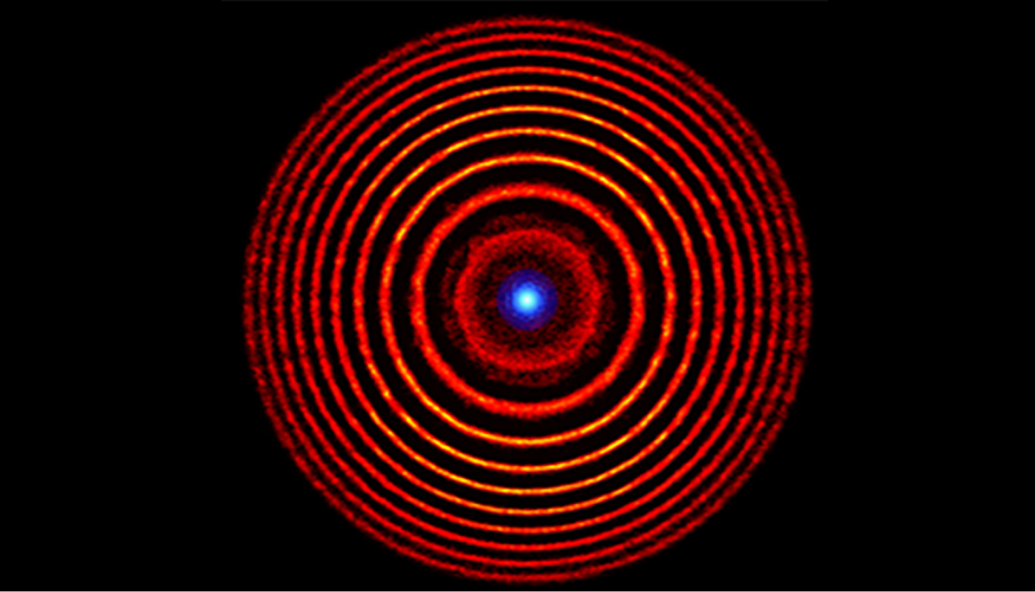
Thomas Schenkel
Lawrence Berkeley National Laboratory
The presenter discussed recent experiments at the BELLA petawatt laser that expanded its focus on electron acceleration to also include ion acceleration and laser-matter interactions with solid targets. The 1-Hz repetition rate of BELLA allows parametric explorations with thousands of shots. One example is nuclear reactions in plasmas and moderately hot targets, where unreasonably high values of apparent electron screening potentials have been observed in studies of light ion fusion that now can be investigated with higher precision. Pulsed ion beams provide access to the time domain of ion–solid interactions, and they have been used to drive materials very far from equilibrium. The talk reported on studies of color center qubit formation and damage accumulation in semiconductor devices with intense, pulsed ion beams.
Thomas White
University of Nevada, Reno
The study of warm dense ma...
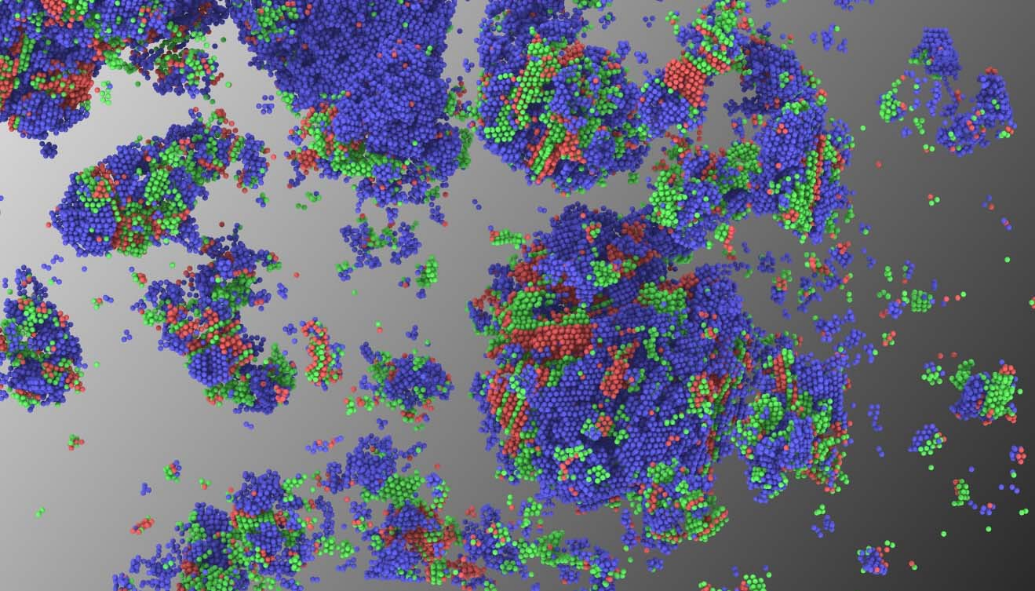
Thomas White
University of Nevada, Reno
The study of warm dense matter (WDM) has important applications, such as understanding controlled thermonuclear fusion and in material processing. Laboratory experiments are now able to create WDM states with a range of techniques, allowing critical tests of theory and modelling. Still, the experimental possibilities to diagnose dense matter are rather limited. Recent advances in free-electron laser technology have produced x rays with a high enough flux in a small enough bandwidth to allow the properties of WDM to be probed on a shot-by-shot basis. The presenter provided an overview of x ray scattering experiments, along with preliminary results of an experiment principally designed to measure the ion-ion dynamic structure factor and sound speed in warm dense methane. Some of these results were also compared to results from quantum and classical atomistic simulations.
Scott Bergeson
Brigham Young University
The presenter discussed hi...
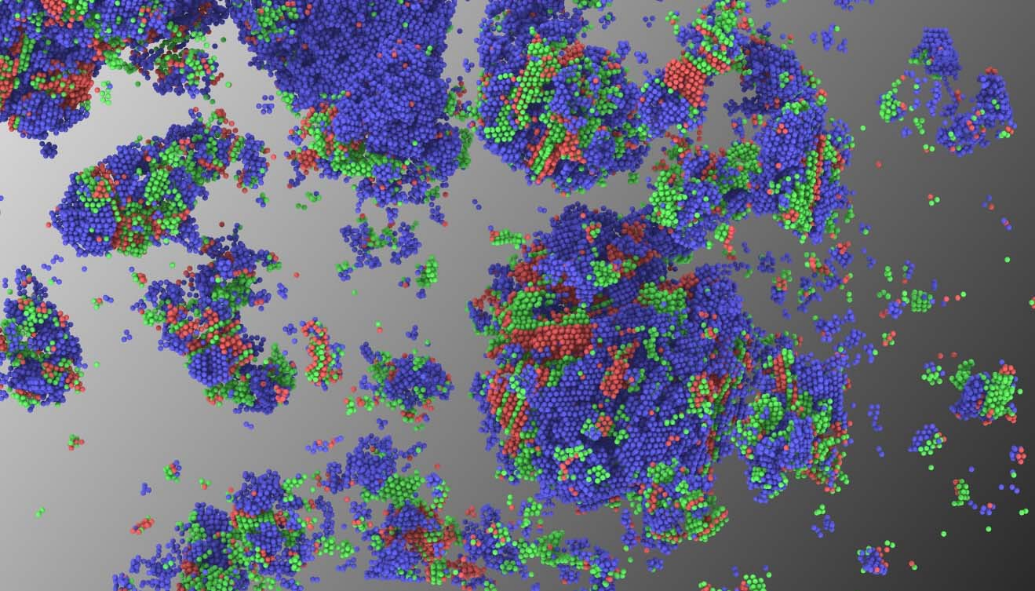
Scott Bergeson
Brigham Young University
The presenter discussed his research aimed at understanding ion-ion interactions in a multi-species, strongly coupled environment. Ion mass provides spatial confinement in high-density, laser-driven implosions of solid ices. Careful modeling of the target, as well as the laser profile and precision cryogenic targetry, are required to create targets of the right size, shape, structure, and density to optimize fusion yield. These efforts attempt to balance the competing demands of temperature, density, and size requirements for achieving self-sustained nuclear burn. Inertial confinement occurs as a competition between the ion response to the field generated by the other charges in the plasma (expansion) and the friction forces generated as distributions of ions move past each other (confinement). The presenter’s research team designed experiments to examine aspects of this confinement using a high-energy-density-plasma simulator.
Frontiers of high-pressure research at the European Synchrotron Radiation Facility
June 6, 2019
...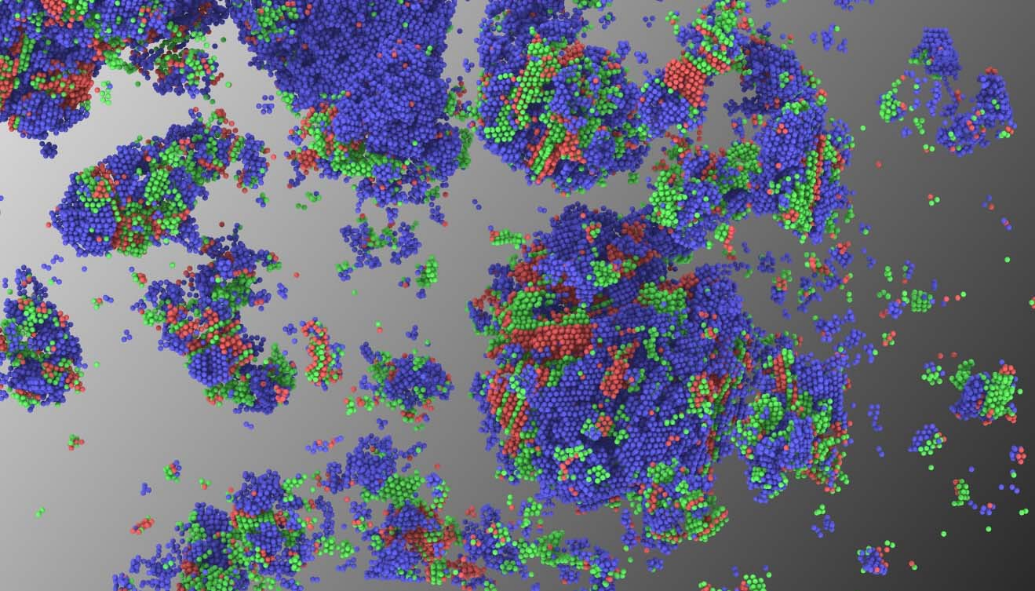
Frontiers of high-pressure research at the European Synchrotron Radiation Facility
June 6, 2019
Sakura Pascarelli
European Synchrotron Radiation Facility (ESRF)
The presenter provided an overview of static and dynamic compression activities and recent scientific results obtained at the ESRF. She also discussed future projects and the unique science opportunities offered by ESRF’s Extremely Brilliant Source (EBS), which will be operational in 2020. The EBS will offer significantly higher flux density and higher coherence, leading to important perspectives for extreme matter studies. She described three EBS-related projects: (1) a high-flux, nano-X-ray, diffraction beamline for science at extreme conditions; (2) pushing the limits of nuclear resonant scattering in energy and spatial resolution; and (3) a high brilliance EXAFS beamline optimized for time resolved and extreme conditions applications.
Kenneth Wharton
San Jose State University
Despite the fact that Be...
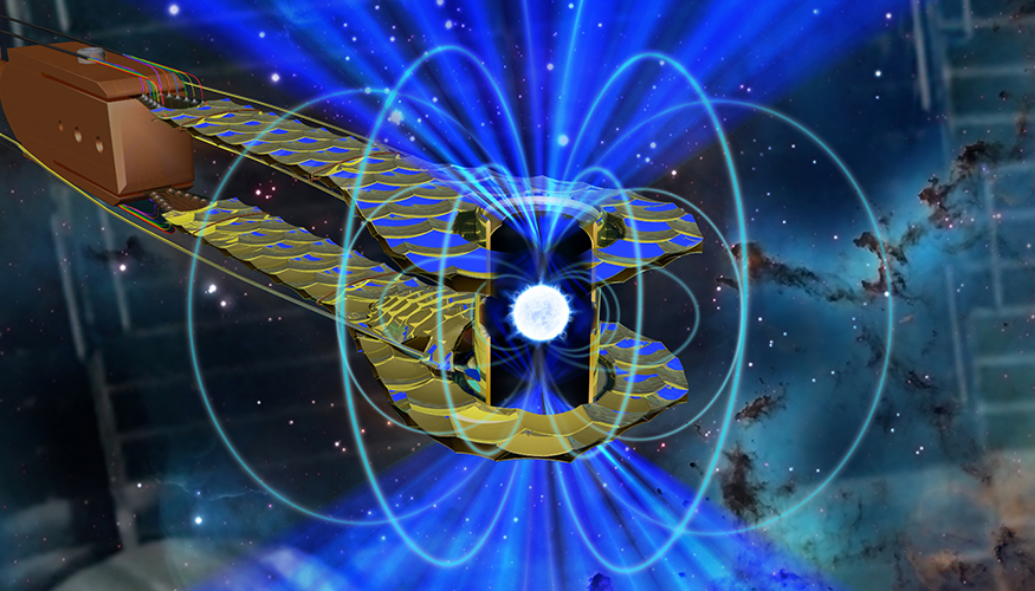
Kenneth Wharton
San Jose State University
Despite the fact that Bell’s Theorem tells us something profound about our universe, too many physicists dismiss it as a reconfirmation of something we already knew. Many others have a misapprehension of the main result, incorrectly believing that it rules out hidden variables or does not apply to probabilistic models. And even experts in quantum foundations are sometimes unaware of subtleties concerning the role of an “arrow of time” in Bell’s analysis, and an associated time-symmetric option for modeling entanglement phenomena in spacetime. This talk attempted to clarify these and other issues, surveying Bell’s Theorem and its fascinating implications.