The Center invites HED science researchers to deliver presentations as part of our weekly seminar series for LLNL staff, postdocs, and interns.
All presentations are the work of the speakers and owned by their respective institutions. We thank the speakers for permission to post their work here. To watch featured seminars, please visit the Livermore Lab Events YouTube channel.
If you are interested in delivering a seminar, we invite you to contact Federica Coppari, seminar series chair, at coppari1 [at] llnl.gov (coppari1[at]llnl[dot]gov)
Julia Mikhailova
Princeton University
Plasma optics have emerged as an a...
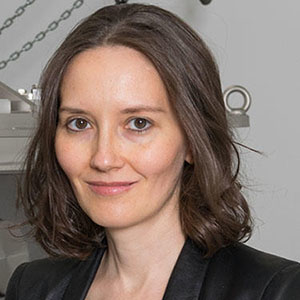
Julia Mikhailova
Princeton University
Plasma optics have emerged as an attractive toolkit to manipulate high-intensity light. Relativistic plasma mirrors can generate high-order harmonics of petawatt lasers with theoretical energy-conversion efficiencies exceeding 60%. This seminar will provide an overview of our work revealing the fundamental processes behind the efficient frequency up-conversion by plasma mirrors. We will discuss our recent experiments in which we have generated high-order harmonics in the relativistic regime using the laser beams from our 20-terawatt, 25-femtosecond-pulsed system focused onto solid targets. We will further examine remaining challenges in experimental methods and opportunities relating to driving waveform optimization.
Luke Stanek
Department of Computational Mathematics, Science and Engineering
Michigan S...
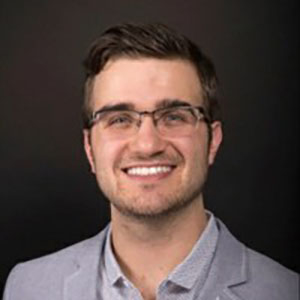
Luke Stanek
Department of Computational Mathematics, Science and Engineering
Michigan State University
Hydrodynamic models rely on closures via equations of state and transport coefficients. Due to the lack of experimental data for transport coefficients, we heavily rely on molecular dynamics simulations. The key input to these simulations is the force law, the choice of which can vary the computational cost by orders of magnitude.
In this talk, we discuss two complementary approaches: determining a computationally efficient, yet acceptable force law, and optimally using sparse transport coefficient data. We delineated the accuracy boundary of force laws by extracting force-matched pair-interaction potentials from Kohn-Sham molecular dynamics data. These pair-interaction potentials reduce the amount of computational resource needed by orders of magnitude allowing for large-scale simulations. Our results rule out the need for three-body potentials above specific element dependent temperatures. These conclusions highlight that accurate results can be obtained in different regimes using disparate methods. We discuss how machine learning provides an avenue to accurately predict transport coefficients with sparse data spanning these regimes. Moreover, we utilize transport coefficient data from computationally cheap models to predict computationally expensive transport coefficients.
Bruce Macintosh
Kavli Institute for Particle Astrophysics and Cosmology
Department of P...
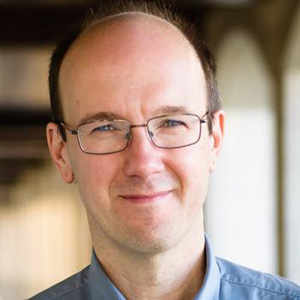
Bruce Macintosh
Kavli Institute for Particle Astrophysics and Cosmology
Department of Physics, Stanford University
More 4,000 extrasolar planets are now known, but almost all have been detected through indirect methods—measuring the parent star’s Doppler shift or brightness variations. Direct detection refers to spatially separating the planet’s light from that of the star. It is extremely challenging—Jupiter in our solar system is 10-9 the luminosity of the sun—but allows observations of planets inaccessible to other methods, particularly the outer parts of target systems, and allows spectral a characterization of a planet’s atmospheric properties.
I will discuss the optical physics that makes direct detection challenging, and the techniques—adaptive optics, coronagraphy, and image processing—that can overcome these challenges. To date, direct detection has been successful for young Jupiter-like planets, and I will show highlights of those discoveries, including those from the LLNL-led Gemini Planet Imager.
Burkard Militzer
Department of Earth and Planetary Science, University of California, Berkeley
...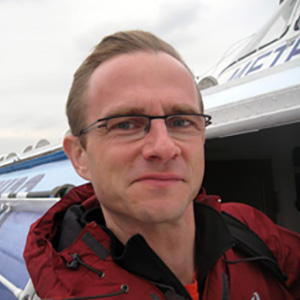
Burkard Militzer
Department of Earth and Planetary Science, University of California, Berkeley
This talk will discuss two equation of state applications. First, we will describe a first-principles equation of state (FPEOS) database for matter at extreme conditions that we put together by combining results from path integral Monte Carlo and density functional molecular dynamics simulations of eleven elements and ten compounds [1]. For all these materials, pressure and internal energy are provided over a wide density-temperature range from 0.5 to 50 g/cc and from 105 to 109 K. Results from 5000 first-principles simulations were combined. In this talk, we focus on isobars, adiabats and shock Hugoniot curves of different silicates in the regime of L and K shell ionization. Second, we will discuss how uncertainties in the equation of state of hydrogen-helium mixtures affect our understanding of giant planets. We will review the gravity measurements of the Juno spacecraft that has been in orbit about Jupiter since 2016. Interpreting these measurements has been a challenge because it is difficult to reconcile the unexpectedly small magnitudes of the moments J4 and J6 with conventional interior models that assume an ab initio equations of state as well as a compact core of ice and rock. We conclude by discussing models for Jupiter’s interior that include cores that have been substantially diluted with hydrogen and helium.
Riccardo Bonazza
Department of Engineering Physics, University of Wisconsin-Madison
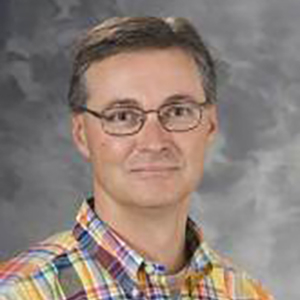
Riccardo Bonazza
Department of Engineering Physics, University of Wisconsin-Madison
The Richtmyer-Meshkov instability (RMI) develops upon impulsive acceleration of the interface between fluids of different acoustic impedance. The RMI consists in the unbounded growth of any perturbations initially present on the interface, and it is a consequence of the baroclinical deposition of vorticity on the interface. Eventually, the interface develops into a turbulent layer leading to the mixing of the two fluids. Flows of this type occur in nature and in man-made settings, over very large ranges of length, time, and energy scales: from experiments for the attainment of inertial confinement fusion (ICF), to supersonic combustion systems, to supernovae explosions. Shock-induced mixing has very negative effects in the case of ICF while it holds great potential to improve the combustion processes in the case of hypersonic engines. Two of the fundamental parameters that govern these phenomena are the Atwood number (measuring the original density contrast) and the Mach number. The experiments described here take place in a vertical shock tube with large, square internal cross section. The initial condition consists of a shear layer between helium and argon. A downward-propagating planar shock wave of Mach number 1.6 or 2.2 accelerates the interface. Measurements of 2-D concentration and velocity fields in a vertical cross section of the flow are obtained using planar laser-induced fluorescence (PLIF) and particle image velocimetry (PIV), respectively. Field statistics and integral quantities are extracted from these data and turbulence and mixing parameters are evaluated. From them, the outer scale Reynolds number is estimated to reach between 10,000 and 20,000 at the late times, indicating that the flow has crossed the threshold of fully developed turbulence.
Michael Rosenberg
Laboratory for Laser Energetics, University of Rochester
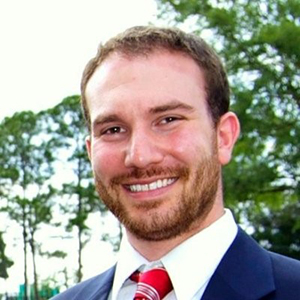
Michael Rosenberg
Laboratory for Laser Energetics, University of Rochester
Laser direct drive is one of three main approaches to achieving inertial confinement fusion ignition and high yield in the laboratory. While cryogenic direct-drive implosions designed to optimize implosion performance are conducted on the 30-kJ OMEGA laser, MJ-scale experiments on the National Ignition Facility (NIF) are critical to understand scale-dependent aspects of direct-drive physics—principally related to laser‒plasma interactions—at ignition-relevant conditions. Spherical-geometry experiments over a range of convergence ratios have shown control of laser energy coupling and implosion symmetry using contoured shells and beam pointing, and wavelength detuning has been used to partially mitigate cross-beam energy transfer. Stimulated Raman scattering has been identified as a primary source of hot electrons and mid-Z layers have been found to reduce hot electron preheat in implosions. Planar and spherical experiments have generated x-ray radiography data to diagnose laser imprint and imprint mitigation using high-Z coatings. Low-convergence implosions are being explored as neutron sources, and a staged set of enhancements to NIF are proposed to allow for increased convergence and yield.
David Turnbull
Laboratory for Laser Energetics, University of Rochester
...
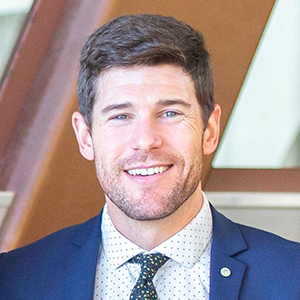
David Turnbull
Laboratory for Laser Energetics, University of Rochester
Plasma heating by inverse bremsstrahlung absorption of electromagnetic radiation was predicted to result in non-Maxwellian electron distribution functions (EDFs) more than 40 years ago, self-consistently modifying the absorption itself. Fokker-Planck simulations suggested that the bulk electrons in such EDFs should be close to super-Gaussian, with an exponent 2<m<5 that is a function of the overlapped laser intensity. In such plasmas, ion-acoustic wave (IAW) frequencies were also shown to increase monotonically with the super-Gaussian exponent, with implications for IAW instabilities including crossed-beam energy transfer (CBET). It was also theorized, however, that the tails of the EDF might deviate from the bulk distribution. Despite the many implications for laser-plasma experiments, these predictions remained largely untested for decades. I will discuss recent experiments at the University of Rochester Laboratory for Laser Energetics that have validated many of these predictions, including the shape of the laser-heated EDFs and their impacts on CBET and absorption.
Chris Pickard
University of Cambridge
High pressure and materials research has been transf...
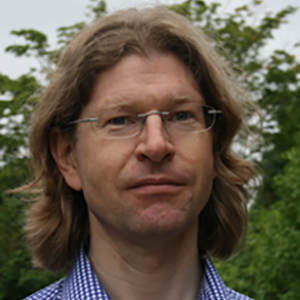
Chris Pickard
University of Cambridge
High pressure and materials research has been transformed by the ability to predict both the structures and properties of materials from first principles. In many cases these predictions have been later confirmed by experiment. In others they have provided fruitful new directions to explore.
This progress has been achieved through the combination of stochastic approaches with reliable and efficient first principles methods. Diverse ensembles of initial structures can be generated, and structurally optimized. The resulting low energy structures are candidates for stable, and metastable, phases and/or defects and interfaces that might be experimentally realized. Success, of course, depends on a sufficiently broad and thorough sampling of configuration space.
A purely random strategy, as employed by Ab Initio Random Structure Searching (AIRSS), is entirely parallel, and a natural fit to the high throughput computation (HTC) paradigm. Challenging cases can be tackled by designing the initial random structures so that they focus the search in regions of configuration space that are anticipated to yield success.
The design of these random “sensible” structures will be explored, along with some new directions which promise to help our understanding of, and accelerate random search, along with applications to high pressure and materials research—from dense hydrides approaching room temperature superconductivity, to surprising astrophysical reactions and complex interfacial materials.
Jonathan Peebles
Laboratory for Laser Energetics, University of Rochester
Over the last de...
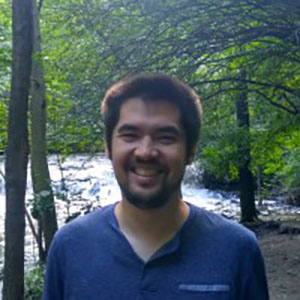
Jonathan Peebles
Laboratory for Laser Energetics, University of Rochester
Over the last decade, magnetic fields generated from a laser-driven current passing through a coil have become increasingly popular. The laser driven coil (LDC) fills a unique role in HED and potentially ICF science by providing magnetic fields to laser facilities without the need for bulky, destructive pulsed power devices, which are often incompatible with lasers due to debris. B-dot probes, Faraday rotation, and transverse (perpendicular to the coil axis) proton radiography have been used in previous LDC experiments, where fields of 1 kilo-Tesla over a millimeter cubed volume are commonly cited. However, in the body of recent work studying these coils, kilo-Tesla level fields are not the only measurements that have been made. Fields from LDCs vary over several orders of magnitude with different designs and drivers; in some cases, the same experiment has magnetic field measurements that differ by close to an order of magnitude depending on which diagnostic is being used.
To clarify the results of previous work, and to gain an understanding of which diagnostics are more reliable in this regime, we performed LDC experiments on OMEGA-EP using all of the aforementioned diagnostics, and have introduced a new technique called axial proton probing. The diagnostic responses for the experiment were compared with those for a known magnetic field. Not only was a consistent result found between the four primary diagnostics, but the results provided information on the diagnostics themselves, and some additional insight that may help explain the findings of other previous experiments.
Martin Greenwald
MIT Plasma Science & Fusion Center
The SPARC tokama...
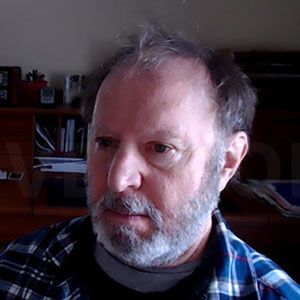
Martin Greenwald
MIT Plasma Science & Fusion Center
The SPARC tokamak is currently under design as a mid-sized, deuterium-tritium (DT) burning magnetic confinement experiment. By employing novel high-temperature superconducting magnets, it will operate at 12.2 T in a device of a size and configuration similar to many experiments that have been deployed in the world’s fusion research program. Operation at high field will however, allow SPARC to be the world’s first experiment to create and confine a plasma that produces net fusion power. The performance to satisfy that mission has been defined as a fusion gain, Q > 2 and PFusion > 50 MW which would be comfortably more than the 25 MW of RF input power. Achieving this goal, we believe, would be a sufficient demonstration to place fusion firmly into the world’s energy plans. Significant margin against uncertainties in performance assumptions has been built into the design such that well-established physics predicts that SPARC could produce more than 140 MW of fusion power with Q > 10. Successful operation of SPARC would inform and enable the construction of a fusion pilot plant—a device with a major radius of about 3 m, producing over 500 MW of fusion power.
Sebastien Merkel
Université de Lille?CNRS
Microstructures define the arrangement of elemen...
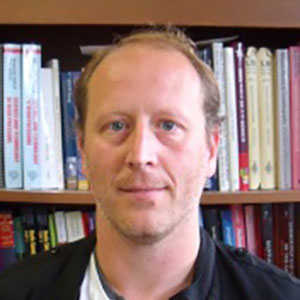
Sebastien Merkel
Université de Lille?CNRS
Microstructures define the arrangement of elements within a material, including phase distributions, grain sizes or grain orientations, and play a key role in defining materials properties. In the geosciences, microstructures affect the propagation of seismic wave and can be used to infer dynamic processes deep inside the Earth based on seismic observations. In materials science, microstructures affect the material’s mechanical properties, such, as strength. Phase transformations and plastic deformation can affect microstructures in a material and both effects can be studied under extreme conditions. In this seminar, I will show how new experiments can be used to study microstructures and the underlying physical mechanisms, both in static and dynamic experiments.
In the first part of the presentation, I will look at phase transformations in minerals. I will present the technique of multigrain crystallography in the diamond anvil cell and how it can be used for follow the distribution of phases, number of grains, and grain orientations in materials undergoing phase transformation and deformation, with applications in the field of geophysics. In the second part of the presentation, I will show how new designs of laser-driven compression experiments coupled with X-ray free electron lasers that can be used to study stress and texture in polycrystals at extreme strain rates. I will show how the technique can be used along with results on the hcp phase of Fe.
Brian Spears
Lawrence Livermore National Laboratory
Large-scale scientific endeavors often...
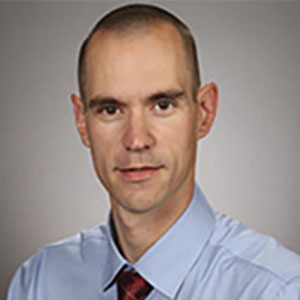
Brian Spears
Lawrence Livermore National Laboratory
Large-scale scientific endeavors often focus on improving predictive capabilities by challenging theory-driven simulations with experimental data. Yet, both simulation and experiment have become overwhelmingly rich, with a complex of observables including scalars, vector-valued data, and various images. At Lawrence Livermore National Laboratory (LLNL), we are using modern artificial intelligence (AI) technologies to combine predictive simulation models with rich experimental data. We call this set of methods cognitive simulation. We will describe a strategic LLNL research effort aimed at using recent advances in deep learning, computational workflows, and computer architectures to develop improved predictive models.
We will present work from a wide range of applications, including using inertial confinement fusion research and experiments at the world’s largest laser, the National Ignition Facility (NIF), as a testbed. We will describe advances in machine learning architectures and methods necessary to handle strong nonlinearities and multimodal data in ICF science and other applications. We will also cover state-of-the-art tools that we developed to steer physics simulation, uncertainty quantification, and model training at enormous scale. We finally describe our ongoing efforts to combine these technologies to support a broad spectrum of Lab science applications. We will especially emphasize development of self-driving, high-repetition-rate lasers as a prototype for embedding AI technologies in next-gen experimental facilities.
Suzanne Ali
Lawrence Livermore National Laboratory
It is important to a ...
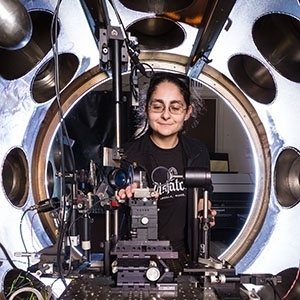
Suzanne Ali
Lawrence Livermore National Laboratory
It is important to a number of fields to be able to accurately simulate processes and material responses under extreme conditions. Using sophisticated hydrodynamic codes, we can model giant impacts, planetary formation, and inertial confinement fusion implosions. The degree to which these simulations reflect reality, however, is dependent on how well we understand the materials and physics involved. Dynamic compression experiments are one of our vital tools for improving our understanding of material properties under extreme conditions, and I will be presenting on the development and status of the Ramp Compression Campaign on the National Ignition Facility. Obtaining high-precision, accurate ramp-compression data on materials under extreme conditions requires a well-validated platform. To date, we have addressed key issues in the development of ramp compression on the NIF: we have demonstrated the ability to ramp-compress metals to high pressure, including copper to ~23 TPa, we have developed analysis tools and experimental design predictive capability, and we have performed cross-platform equation of state (EOS) experiments validating the NIF platform.
Cameron Geddes
BELLA Center, Lawrence Berkeley National Laboratory
Ultra...
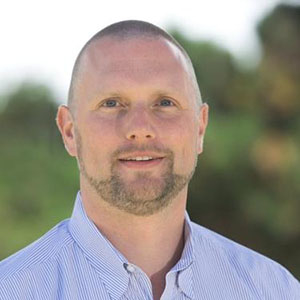
Cameron Geddes
BELLA Center, Lawrence Berkeley National Laboratory
Ultrashort pulse lasers enable resonant excitation of plasma waves, efficiently driving structures that can accelerate particles at rates of in the range of a GeV per centimeter. Guiding of such lasers in plasma structures formed by laser heating, capillary discharges and most recently by combining these two techniques has extended the depth of interaction from millimeter to tens of centimeter scale, enabling energies up to 7.8 GeV to be achieved. Experiments to combine two such stages at multi-GeV energies are being prepared. At the GeV energy scale, compact electron beams are being used to develop novel compact photon sources including free electron lasers, and MeV photons from Thomson scattering. The same laser pulses are being used for acceleration of ions from solid targets using target sheath fields, and experiments are being planned to access more efficient ion acceleration regimes and new frontiers in high energy density science in the near future, supported by a new short focal length capability. While these experiments are proving the building blocks of a future plasma based high energy physics collider and photon sources, laser development holds the key to scaling to the repetition rate and precision required.
Siegfried Glenzer
SLAC National Accelerator Laboratory
Stanford University &...
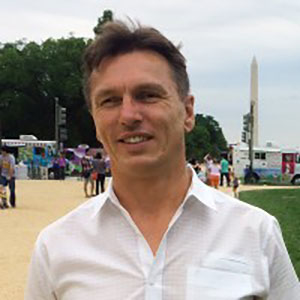
Siegfried Glenzer
SLAC National Accelerator Laboratory
Stanford University
Normally, what surrounds us are gases, liquids, or solids. But elsewhere in the universe, 99% of the observable matter exists under extreme conditions that lead to exotic states of matter and the formation of plasmas and warm dense matter. Specifically, near the center of Jupiter, hydrogen becomes liquid or even solid - a process important to understand the evolution of our solar system. In the center of the sun, hydrogen is a plasma that burns itself up by nuclear fusion - a process humans want to harness for clean energy production on earth. In the mantle of Neptune, hydrogen and carbon cannot mix and are postulated to form giant diamonds – a process that can explain Neptune’s excess heat generation. On the other hand, very hot plasmas are postulated to eject particles that we can observe as cosmic rays and that are a million times more energetic than mankind’s largest machines. At SLAC, we are now studying these extreme states of matter in the laboratory. We apply enormous pressures to earthbound samples and use our X-ray laser, the Linac Coherent Light Source, to take split-second photographs of the states that result. This lecture will describe these experiments. The information we are gathering provides fundamental insights into the physical properties of matter in extreme conditions whose understanding is important for modeling astrophysical processes and for pursuing controlled fusion. To further advance this field, a new upgrade has been proposed to bring state-of-the art lasers to the LCLX X-ray beam and which has recently been reviewed and endorsed by the US user community.
Matthew Foulkes
Imperial College London
Given access to accurate solutio...
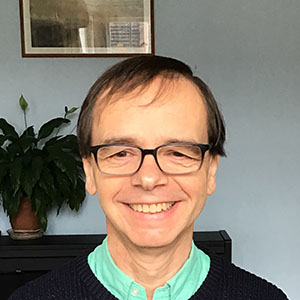
Matthew Foulkes
Imperial College London
Given access to accurate solutions of the many-electron Schrödinger equation, much of chemistry could be derived from first principles. Exact wavefunctions of interesting chemical systems are out of reach because they are NP-hard to compute in general, but approximations can be found using polynomially-scaling algorithms. The key challenge for many of these algorithms is the choice of approximate or “trial” wavefunction, which must balance efficiency versus accuracy. Neural networks have shown impressive power as accurate practical function approximators and promise as a compact trial wavefunctions for spin systems, but problems in electronic structure require wavefunctions obeying Fermi-Dirac statistics. Here we introduce a new deep learning architecture, the Fermionic Neural Network, as a powerful trial wavefunction for many-electron systems. FermiNet is able to achieve accuracy beyond other variational quantum Monte Carlo trial wavefunctions for a variety of atoms and molecules. Using no data other than atomic positions and charges, we predict the dissociation curves of the nitrogen molecule and hydrogen chain, two challenging strongly-correlated systems, to significantly higher accuracy than the coupled cluster method, widely considered the most precise scalable method for quantum chemistry at equilibrium geometry. This demonstrates that neural-network wavefunctions can improve the simple and appealing variational quantum Monte Carlo method to the point where it outperforms much more complex ab-initio quantum chemistry methods, opening the possibility of accurate direct optimization of wavefunctions for previously intractable molecules and solids.
Steve MacLaren
Lawrence Livermore National Laboratory
In inertial confin...
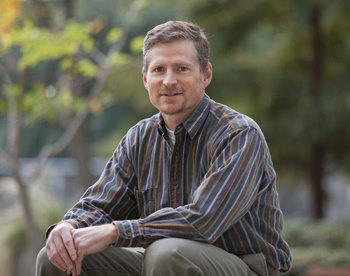
Steve MacLaren
Lawrence Livermore National Laboratory
In inertial confinement fusion, the threshold for ignition is a highly dynamic quantity as the sources and sinks of power in the hot spot can vary rapidly. In this talk, we consider the ignition condition as a race between heating and disassembly rates and combine this ansatz with a prior solution to the fusion hot-spot thermodynamics to develop a Lawson-like ignition criteria for pressure X confinement time (p-tau) versus temperature. Low-Z capsule designs reach the temperature for this threshold using as much of the shell as feasible as ablator but then are limited in confinement time due to low stagnated mass. An alternate approach, the pushered single shell (PSS) introduces a dense inner layer of Mo-Be alloy that is smoothly graded outward to pure Be, increasing the confinement time at stagnation and lowering the temperature requirement at the ignition threshold. We describe both the design of and initial experimental results from this type of capsule.
Brian Appelbe
Imperial College London
A burning thermonuclear plasma is a unique plasma en...
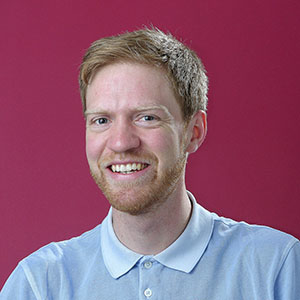
Brian Appelbe
Imperial College London
A burning thermonuclear plasma is a unique plasma environment. It is characterized by extremely large temperatures, densities and fluxes of α particles and radiation that evolve over very short temporal and spatial scales. A key question in ICF is how the transport processes accompanying these conditions affect the achievable yield. Furthermore, the effects of a magnetic field in a burning plasma deserve attention due to current research into magnetically-assisted ICF and Magneto-Inertial Fusion schemes, and the possible presence of self-generated magnetic fields in ICF experiments.
This talk summarizes results from a theoretical and computational investigation of burning plasmas, with and without magnetic fields, with a particular focus on two novel results. Firstly, it is shown that the interaction of a flux of α particles with a thermal plasma can induce an electronic current in the plasma. This current leads to new transport processes in burning plasmas. Secondly, the presence of a magnetic field in a propagating thermonuclear burn wave can lead to the formation of transport barrier at the burn front, reducing the rate of burn propagation.
Tammy Ma
Lawrence Livermore National Laboratory
The Advanced Photo...
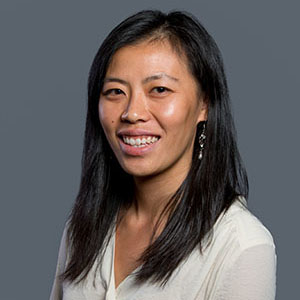
Tammy Ma
Lawrence Livermore National Laboratory
The Advanced Photon Technologies (APT) high-intensity HED science program at LLNL seeks to provide new capability in, and drive forward frontier HED with short-pulse laser science and applications. We will discuss recent work to develop laser-driven sources for DOE applications, including multi-ps, very high energy short pulse laser experiments on the NIF-ARC laser; and describe work towards a closed-loop paradigm to accelerate scientific discovery, integrating high-repetition-rate laser experiments, machine learning, and physics-based cognitive simulations. Using these novel tools, areas of active research include high-intensity laser interactions with matter, laser-driven secondary sources, plasma optics, future light sources, and the development of high-throughput diagnostics, targetry, modeling, and machine learning. Finally, we will touch on efforts to develop a purposeful set of partnerships and engagements to better leverage both national and international HED efforts.
Louise Willingale
University of Michigan
Laser-plasma interactions produce strong temperature and ...
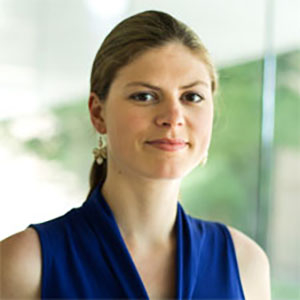
Louise Willingale
University of Michigan
Laser-plasma interactions produce strong temperature and density gradients that generate megagauss strength magnetic fields through the Biermann-battery effect. We performed experiments using the OMEGA EP laser system and used proton radiography to measure the strength, spatial profile, and temporal dynamics of the self-generated magnetic fields. The target material was varied and the plastic (CH), aluminum, copper, or gold targets exhibited different magnetic field structures. Mid-Z targets had two distinct regions of magnetic field, one produced by gradients from electron thermal transport and the second from radiation-driven gradients. Extended magnetohydrodynamic simulations including radiation transport reproduced the key experimental features, including the magnetic field generation and double ablation front formation.
Bruce Remington
Lawrence Livermore National Laboratory
Highlights from the NIF Discovery Science p...
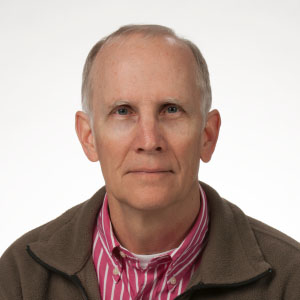
Bruce Remington
Lawrence Livermore National Laboratory
Highlights from the NIF Discovery Science program will be presented. Examples include nuclear reactions relevant to stellar nucleosynthesis; equations of state at high pressures relevant to planetary interiors; and white dwarf envelopes; Rayleigh-Taylor instabilities relevant to supernovae and supernova remnant evolution; relativistically hot plasmas and target normal sheath acceleration of protons on NIF ARC; magnetic reconnection at high energy densities; and high velocity interpenetrating plasmas that generate collision-less astrophysical shocks, magnetic fields, and accelerate particles relevant to cosmic ray generation.
Marcus Brüggen
Universität Hamburg
The new generation of radio interferometers, such as MeerKAT, A...

Marcus Brüggen
Universität Hamburg
The new generation of radio interferometers, such as MeerKAT, ASKAP, and LOFAR, as well as the recently launched X-ray observatory eROSITA provide a new view of the high-energy Universe. They deliver new insights into a large range of astronomical phenomena, such as active galactic nuclei, cosmic rays and magnetic fields, galaxy clusters, the Warm-Hot Intergalactic Medium, and Dark Energy. In my talk I will present new results from on-going radio surveys and the first data from the Russian-German space observatory eROSITA. I will focus on my work on galaxy clusters and the filaments that connect them, cosmic magnetic fields, and particle acceleration in cosmological structures.
Simon Blouin
Los Alamos National Laboratory
White dwarfs are burned-out stars condemned to a slow ...
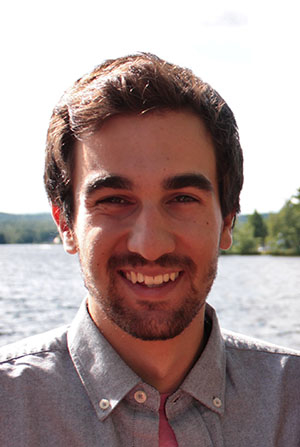
Simon Blouin
Los Alamos National Laboratory
White dwarfs are burned-out stars condemned to a slow cooling that extends over billions of years. Thanks to this simple evolution, it is relatively easy to measure their ages, making them useful cosmic clocks to study the history of our Galaxy. Eventually, the dense C/O plasma that makes up their cores becomes so correlated that it freezes. This process releases latent heat as well as gravitational energy due to the sedimentation of the O-enriched solid. This new energy source temporarily slows the cooling of the white dwarf, and it is important to precisely model it if those stars are to be used as accurate cosmic clocks. Both the melting temperature and the importance of O sedimentation depend on the exact shape of the C/O phase diagram.
To obtain an accurate version of this phase diagram, we have developed a new technique to compute the melting curves of multi-component plasmas. It is based on the Gibbs-Duhem integration technique and semi-grand canonical Monte-Carlo simulations of the liquid and solid phases of the screened, partially relativistic, fully ionized mixture. This approach is 200 times less costly than the competing technique where two-phase molecular dynamics simulations are used, thus allowing a finer sampling of the phase diagram and a vastly superior mitigation of finite-size effects. Our results lead to an improved match between white dwarf cooling models and astronomical observations, and highlight the role played by minor species in white dwarf evolution. This now well-tested method also presents an interesting potential for non-astrophysical systems (e.g., alloys).
Bingqing Cheng
University of Cambridge
A central goal of computati...
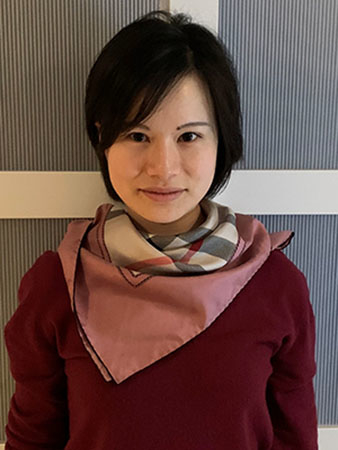
Bingqing Cheng
University of Cambridge
A central goal of computational physics and chemistry is to predict material properties using first principles methods based on the fundamental laws of quantum mechanics. However, the high computational costs of these methods typically prevent rigorous predictions of quantities at finite temperatures, such as chemical potential, heat capacity, and thermal conductivity.
In this talk, I will first discuss how to enable such predictions by combining advanced statistical mechanics with data-driven machine learning interatomic potentials. As an example, for the omnipresent and technologically essential system of water, a first-principles thermodynamic description not only leads to excellent agreement with experiments, but also reveals the crucial role of nuclear quantum fluctuations in modulating the thermodynamic stabilities of different phases of water. As another example, we simulated the high-pressure hydrogen system with converged system size and simulation length, and found, contrary to established beliefs, supercritical behavior of liquid hydrogen above the melting line.
Besides the computation of thermodynamic properties, I will talk about transport properties, such as a proposed method to compute the heat conductivities of liquid just from equilibrium molecular dynamics trajectories. During the second part of the talk, I will rationalize why machine learning potentials work at all, and in particular, the locality argument. I'll show that a machine-learning potential trained on liquid water alone can predict the properties of diverse ice phases, because all the local environments characterizing the ice phases are found in liquid water.
Megan Eckart
Lawrence Livermore National Laboratory
X-ray microcalorimeter spectrometers have been...
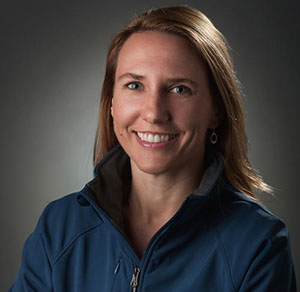
Megan Eckart
Lawrence Livermore National Laboratory
X-ray microcalorimeter spectrometers have been developed for astrophysics space missions, providing imaging arrays with few-eV spectral resolution, but they also offer advantages for use on magnetic fusion energy devices. We recently deployed one of our LLNL/NASA x-ray microcalorimeter spectrometers to the Madison Symmetric Torus (MST) facility at the Wisconsin Plasma Physics Laboratory (WiPPL) and recorded x-ray photons emitted by the helium-like Al impurity ions in a majority deuterium plasma. In this talk we’ll introduce microcalorimeter detectors and discuss the diagnostic capability for magnetic fusion energy experiments, highlighting our experimental work toward demonstrating this capability at MST.
Microcalorimeter spectrometers combine the best characteristics of the x-ray instrumentation currently available on fusion devices: high spectral resolution similar to an x-ray crystal spectrometer, and the broadband coverage (from 0.1 keV to above 12 keV) of an x-ray pulse height analysis system. Fusion experiments are increasingly employing high-Z plasma-facing components and require measurement of the concentration of all impurity ion species in the plasma. This diagnostic has the capability to satisfy this need for multi-species impurity ion data and will also contribute to measurements of impurity ion temperature and flow velocity, Zeff, and electron density.
Andrew Longman
University of Alberta
The generation and application of strong ...
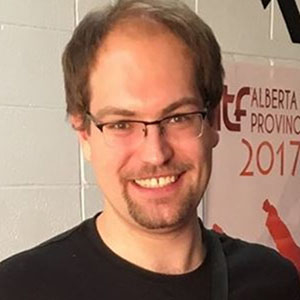
Andrew Longman
University of Alberta
The generation and application of strong (kilo-Tesla) magnetic fields for the study of magnetized ICF, fast ignition, laboratory astrophysics, and particle acceleration/guiding is an area of great and growing interest. In this presentation, we will explore the novel approach for generating kilo-Tesla scale magnetic fields via the inverse Faraday effect driven by lasers carrying angular momentum. The inverse Faraday effect describes the coupling of laser angular momentum directly to plasma electrons and has the unique advantage of not requiring a solid or structured target, making it suitable for both high repetition rate experiments, and in configurations where solid coils are not permissible.
We will numerically explore the inverse Faraday effect in under-dense plasmas with both circularly polarized (CP) beams, and with structured beams carrying orbital angular momentum (OAM). The simulations are carried out using full 3D PIC calculations with Titan-like laser parameters. We demonstrate kilo-Tesla fields extending more than 100 microns in length, and with decay times on the order of picoseconds when driven by 100 femtosecond pulses. We will discuss the advantages and disadvantages of this approach, as well as discussing possible experimental configurations to demonstrate and measure the fields.
Frank Graziani
Lawrence Livermore National Laboratory
The High Energy Density Science Center (HEDS...
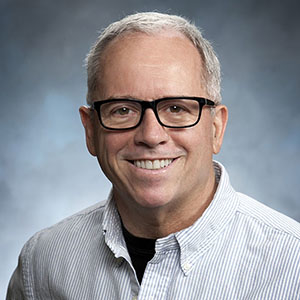
Frank Graziani
Lawrence Livermore National Laboratory
The High Energy Density Science Center (HEDSC) was established in late 2015 through a joint agreement between the Physical and Life Sciences, Weapons and Complex Integration, and NIF and Photon Science directorates. The Center’s goal is to build a high energy density community through support of, and collaboration with, academic partners and to integrate those efforts with the programs at LLNL. The Center is built around four focus areas: (1) education, (2) outreach through workshops and seminars, (3) workforce pipeline to the programs, and (4) enabling research collaborations between LLNL and academic partners.
In this talk, we will present the year 2020 in review and look at what lies ahead in 2021. The year 2020 was a year of both growth and challenges. 2020 saw growth in education programs with a quarter long course on HED diagnostics offered in collaboration with UCSD in addition to the selection of a new HEDS Center postdoctoral fellow. Besides educating the next generation of HED scientists, the HEDS Center continues training students through internships and thesis opportunities. Our outreach and collaboration activities continue through a vibrant seminar series, university partnerships, a planned sabbatical program and visits to community colleges and undergraduate institutions. Inclusion and diversity are an important aspect of our mission and the Consortium for HEDS (FAMU, UC Merced, Morehouse and LLNL) received new funding from NNSA. The Consortium’s goal is to provide research and education opportunities in HEDS for under-represented groups and thereby increase diversity in the field. With the arrival of COVID and working off-site, the HEDS Center had to react to the change. The summer internship program continued, on-line. The sabbatical program and visits to community and undergraduate colleges was put on hold and instead, the Center decided to produce a series of HEDS educational videos for the public.
In 2021 we hope to bring back the sabbatical program and outreach visits to K-12 and community and undergraduate colleges. A call for a new HEDS Fellow postdoc position is out and more HED education programs are planned. In 2021 we will continue to foster international collaboration in HEDS, bring on another thesis student from FAMU and continue the internship program.
Dean Rusby
Lawrence Livermore National Laboratory
High Intensity laser interactions produce a high...
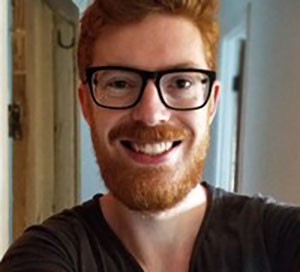
Dean Rusby
Lawrence Livermore National Laboratory
High Intensity laser interactions produce a high current beam of electrons that can be used for many applications. One such applications is the production of a high energy and bright x-ray source via bremsstrahlung. These x-rays possess several qualities, such as pulse durations and high spatial resolutions, that make them ideal for radiography applications. The key to making the brightest x-ray source is converting as much energy as possible from the laser into high energy electrons, which often requires the highest intensity lasers. We have developed an alternative method using a novel target design, the compound parabolic concentrator (CPC) that can be used to confine, focus, and couple more laser energy into the electrons. Experimental measurements have been performed that demonstrate the capabilities of CPCs targets and show that they can produce electrons and x-rays that far outperform planar targets. We also demonstrate the enhancement in x-ray radiography in the MeV regime.
Joyce Guzik
Los Alamos National Laboratory
In 2004, improved analyses of solar spectra resulted in...
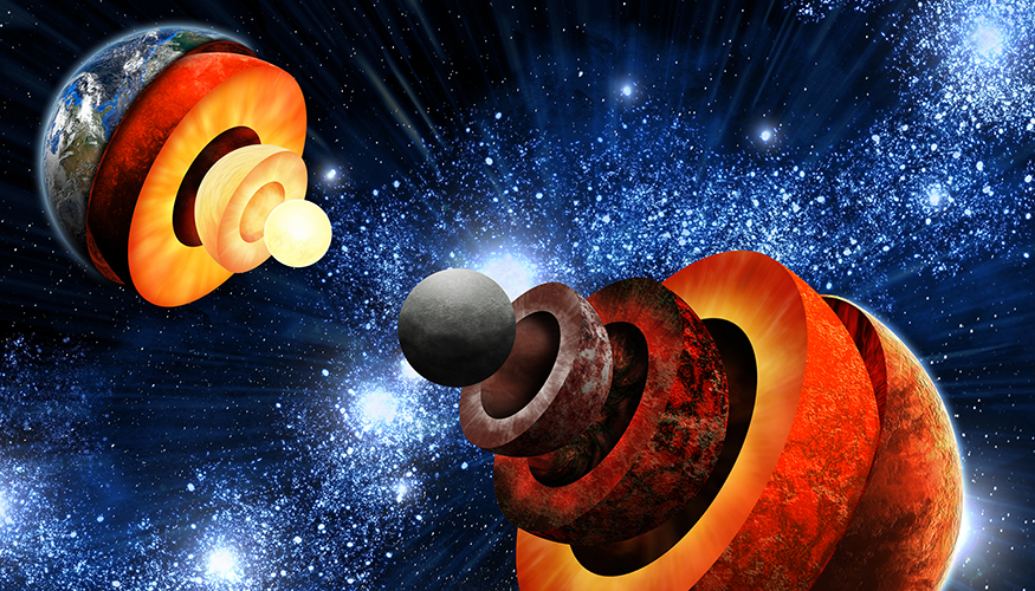
Joyce Guzik
Los Alamos National Laboratory
In 2004, improved analyses of solar spectra resulted in a downward revision of the abundances of elements heavier than hydrogen and helium, in particular, the elements C, N, and O. Solar models evolved using these lower abundances showed discrepancies with inferences from solar oscillation observations (helioseismology). This problem has not been solved satisfactorily in the intervening 16 years. How serious/important is this problem?
This talk will give an overview of how standard solar models are calculated and of the constraints from helioseismology. The talk will also present results of some attempts to change input physics or assumptions in the standard solar model, including opacity modifications, to try to resolve the discrepancies. I would like to have a discussion with the participants after the talk about which directions appear most promising to resolve the problem.
Michael Demkowicz
Texas A&M University
Solids are subjected to bombardment by high-energy, mas...
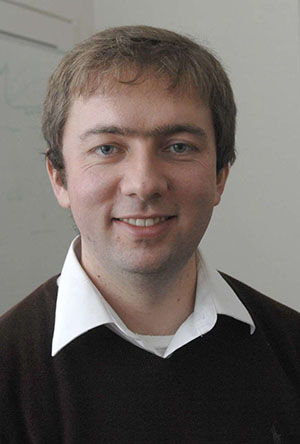
Michael Demkowicz
Texas A&M University
Solids are subjected to bombardment by high-energy, massive particles (electrons, neutrons, ions) in a wide range of energy, weapons, and astrophysical scenarios. I will summarize the fundamental mechanisms by which solids—especially metals—respond to such irradiation, from the dissipation of the particle’s energy in the course of a collision cascade to the generation and subsequent evolution of damage in the material. I will then present a selection of recent research on radiation response—carried out primarily using molecular dynamics simulations—focusing on amorphous and nanocomposite metals. I will conclude with a discussion of prospects for developing novel, radiation-resistant materials based on insights gained from this research.
John Cary
University of Colorado, Tech X
Speed-limited particle-in-cell ...
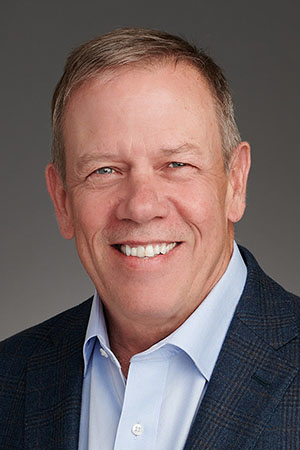
John Cary
University of Colorado, Tech X
Speed-limited particle-in-cell (SLPIC) is an algorithm for achieving orders of magnitude speedup of simulations in cases where kinetic electron effects are important, yet the motion is slow compared with the electron thermal velocity. This is the case for nearly all low-frequency plasma dynamics, but it is especially so for low-temperature plasma discharges where (1) the ions are cold compared with the electrons and (2) the ions are comparatively even more massive, e.g., in Argon discharges. This method has several quirks, including the need to have both a charge and a current weight. This method has now been generalized to include collisions and secondary emission, thereby allowing it to be applied to determine the Paschen curve in irregular geometries. The theory of this new algorithm will be presented along with results. In addition, there will be a few remarks on other subjects, such as device computing and user-friendly code, that contribute to reducing time to solution.
Katie Lewis
Lawrence Livermore National Laboratory
Scientific simulations have driven computing at...
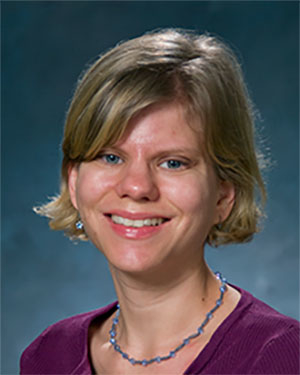
Katie Lewis
Lawrence Livermore National Laboratory
Scientific simulations have driven computing at Lawrence Livermore National Laboratory (LLNL) for decades. During that time, we have seen significant changes in hardware, tools, and algorithms. Today, data science, including machine learning, is one of the fastest growing areas of computing, and LLNL is investing in hardware, applications, and algorithms in this space. While the use of simulations to focus and understand experiments is well accepted in our community, machine learning brings new challenges that need to be addressed. I will explore applications for machine learning in scientific simulations that are showing promising results and further investigation that is needed to better understand its usefulness.